Advances in Animal and Veterinary Sciences
Research Article
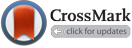
Evaluation of In Vitro Nutrient Digestibility, Fermentation Characteristics and Methane Production of Agro-industrial Byproducts-based Complete Feed Block Treated with Mixed Microbes
Budi Santoso1*, Trisiwi Wahyu Widayati1, Marlyn Nelce Lekitoo1, Bambang Tjahyono Hariadi1, Hermawaty Abubakar2
1Department of Animal Science, Faculty of Animal Science, University of Papua, Manokwari, West Papua 98314, Indonesia; 2Department of Biology, Faculty of Mathematics and Natural Science, University of Papua, Manokwari, West Papua 98314, Indonesia.
Abstract | This study was undertaken to evaluate in vitro nutrient digestibility, fermentation characteristics and methane production of agro-industrial byproducts-based complete feed block treated with mixed microbes. Five treatments were A:complete feed block without microbe (control); B: complete feed block containing 1.5% L. plantarum,1.5% S. cerevisiae and 1% P. aeruginosa; C: complete feed block containing 1.5% L. plantarum,1.5% S. cerevisiae, 2% P. aeruginosa and 2% A. baumanni; D: complete feed block containing 1.5% L. plantarum, 1.5% S. cerevisiae, 1% P. aeruginosa and 1% A. baumanni; E: complete feed block containing 1.5% L. plantarum, 1.5% S. cerevisiae, 2% P. aeruginosa and 2% A. baumanni. Lactic acid bacteria, yeast and cellulolytic bacteria were added to the feed block at 106-108 cfu/g. Results showed that addition of cellulolytic bacteria in feed block reduced (P<0.01) ADF content. Feed blocks containing mixed microbes (B, C, D and E) had higher (P<0.01) acetic acid concentration compared to control feed block (A). Feed block E had the highest N-NH3 and total VFA concentrations than other feed block. Methane production reduced (P<0.01) by 16.2%, 37.6% and 37.6% for feed block C, D and E, respectively when compared to feed block A. Therefore, combination of mixed microbes in the complete feed block decreased fiber fraction contents and methane production as well as improved in vitro fermentation activity.
Keywords | By-products, Feed block, Fermentation, Microbe, Rumen
Editor | Kuldeep Dhama, Indian Veterinary Research Institute, Uttar Pradesh, India.
Received | May 06, 2018; Accepted | June 03, 2018; Published | June 28, 2018
*Correspondence | Budi Santoso, Department of Animal Science, Faculty of Animal Science, University of Papua, Manokwari, West Papua 98314, Indonesia; Email: b.santoso@unipa.ac.id
Citation | Santoso B, Widayati TW, Lekitoo MN, Hariadi BT, Abubakar H (2018). Evaluation of in vitro nutrient digestibility, fermentation characteristics and methane production of agro-industrial byproducts-based complete feed block treated with mixed microbes. Adv. Anim. Vet. Sci. 6(6): 258-264.
DOI | http://dx.doi.org/10.17582/journal.aavs/2018/6.6.258.264
ISSN (Online) | 2307-8316; ISSN (Print) | 2309-3331
Copyright © 2018 Santoso et al. This is an open access article distributed under the Creative Commons Attribution License, which permits unrestricted use, distribution, and reproduction in any medium, provided the original work is properly cited.
Introduction
Livestock production in developing countries largely depends on fibrous feeds, mainly crop residues and low quality pasture that are deficient in nitrogen, minerals and vitamins.The utilization of agricultural byproducts for increasing ruminants production has been received greater research attention within the past few decade because of the higher quantities of those byproducts.
Poor palatability and low bulk density apart from low nutritive value are restricting the utilization of the agricultural byproducts as animal feeds. During these scarcity periods, there is need for easily available feed that can meet nutritional requirements at low cost and is easy to transport. Nutritive value of poor quality and bulky roughages can be improved by densified complete feed block (Salem and Nefzaoui, 2003). Complete feed block is comprised of forage, concentrate and other supplementary nutrients in desired proportion capable to fulfill nutrient requirements of animals.The feeding of complete feed block stabilises rumen fermentation, minimises fermentation loss and ensures better ammonia utilisation (Prasad et al., 2001). Moreover, this technology offers a means to increase milk production, decrease in environmental pollutants, increase in income of farmers, decrease in labour requirement andtime for feeding and reduction in transportation cost of straw (Karangiya et al., 2016).
Feed digestion in the rumen is carried out by microbes, thus the type and population of microbes are important factors that affect the digestibility of nutrients. Seo et al. (2010) revealed that micro-organisms such as Lactobacillus, Streptococcus and Enterococcus are commonly used as probiotics for ruminants. Lactobacillus sp. has been used widely as a feed additive in the dairy cattle industry to improve intestinal health, feed conversion efficiency and milk production (Chen et al., 2013). Cao et al. (2011) also reported that lactic acid bacteria increased dry matter digestibility and decreased ruminal methane production. Oyeleke and Okusanmi (2008) reported that Pseudomonas aeruginosa was found in the rumen of cow, sheep and goat approximately 9% of the bacteria species and was able to hydrolyse cellulose. Moreover, Vinotha and Maheswari (2014) revealed that P. aeroginosa isolated from sugarcane bagasse was identified with the help of well zone formation in the cellulose fermentation medium. Chang et al. (2015) noted that Acinetobacter sp. was found in the rumen of Korean native cattle and it was belonged to cellulolytic bacteria. In the previous study, Santoso et al. (2016) concluded that the addition of Lactobacillus plantarum, Saccharomyces cerevisiae, Acinetobacter baumannii and P. aeruginosa in concentrate improved rumen fermentation activity and the digestibility of nutrients in vitro. The present study was therefore designed to evaluate in vitro nutrient digestibilty, fermentation characteristic and methane (CH4) production of agro-industrial byproducts-based complete feed block treated with addition of mixed microbes.
Materials and Methods
Preparation of Complete Feed Block
Rice straw and palm oil frond were collected from paddy field and palm oil plantation areas, respectively in Prafi District, Manokwari regency, Indonesia (longitude 133°48’E and latitude 00°53’S) with a mean altitude of 128 m sea above level. Tofu and cassava wastes were obtained from small-scale food industry located in Manokwari and Prafi Districts.In order to prepare complete feed block, rice straw and palm oil frond were dried under the sun and then chopped with a chopper machine to 1-2 cm lengths. Tofu waste and cassava waste were dried in an oven at 60°C for at least 48 hand were ground to pass through a 1 mm sieve in Wiley mill. L. plantarum was isolated from Pennisetum purpureophoides that has been prepared by Santoso et al. (2013). L.plantarum was cultured using MRS broth at 30 °C for 48 h (Santoso et al., 2016; Santoso et al., 2017), meanwhile S. cerevisiae was cultured using malt extract broth at 30 °C for 48 h (Newbold, 1995). Cellulolytic bacteria i.e P. aeroginosa and A. baumannii were isolated from rice straw and and palm oil seed waste, respectively and were cultured using Carboxymethyl cellulose. The ingredients of feed block were manually mixed by hand and then sprayed on top with a culture of lactic acid bacteria, yeast and cellulolytic bacteria at 106, 108, 106 cfu/g, respectively.
The experiment was arranged in a completely randomized design with five treatments and three replications per treatment. Five treatments were A, complete feed block without microbe; B, complete feed block containing 1.5% L. plantarum,1.5% S. cerevisiae and 1% P. aeruginosa; C, complete feed block containing 1.5% L. plantarum,1.5% S. cerevisiae and 2% A. baumanni; D, complete feed block containing 1.5% L. plantarum, 1.5% S. cerevisiae, 1% P. aeruginosa and 1% A. baumanni; E complete feed block containing 1.5% L. plantarum, 1.5% S. cerevisiae, 2% P. aeruginosa and 2% A. baumanni (Table 1). All complete feed blocks were formulated to be isonitrogenous (12% CP, DM basis). About 500 g of mixed complete feed was transferred into a hydraulic press to makes block of size 15×10×8cm.
Donor Animals and In vitro Rumen Fermentation Characteristics
Rumen fluid, which required for in vitro gas production technique was obtained from two rumen fistulated Ongole crossbreed cattle preconditioned for three weeks with 6.8 kg DM of king grass (P. purpuroephoides) to meet their maintenance requirement.
Rumen liquor was collected with a manual suction apparatus before the morning feeding and strained through four layers of cheese cloth into a pre-warmed thermos flask. In vitro gas production was carried out using the Hohenheim gas test according to procedures of Menke and Steingass (1988) previously described by Santoso et al. (2016) and Santoso et al. (2017). Briefly, oven-dried samples of about 300±5 mg were weighed in triplicate into 100 ml glass syringes (Model Fortune, Häberle Labortechnik, Lonsee-Ettlenschieß, Germany) with pistons that were lubricated with vaseline. Additionally, three parallel syringes that contained mixtures of rumen liquor-buffer without substrate served as blanks.The syringes were incubated in a water bath at 39°C for 24 h, before the addition of 30±1.0 ml of rumen liquor-buffer mixtures into each syringe. Each syringes were incubated in a water bath at 39°C for 48 h and were gently shaken every 8 h. The volume of gas that was released from each syringe was recorded before incubation (0 h) and 1, 2, 4, 6, 8, 12, 24, 36 and 48 h of incubation. To facilitate CH4 measurement, glass syringes fitted with an extra outlet containing gastight septum for gas sampling as previously demonstrated by Santoso et al. (2016). One hundred micro litter of gas was sampled from the headspace of syringe in an airtight syringe at 24 and 48 h of incubation. Methane was determined by injection 100 ml of gas into a chromatograph gas (GC model 263-50, H Hitachi Ltd., Ibaraki, Japan).
Table 1: Ingredients composition (%) of the complete feed block (DM basis)
Ingredients | Experimental Feed Blocks | |||||||
A | B | C | D | E | ||||
Rice straw | 12.0 | 12.0 | 12.0 | 12.0 | 12.0 | |||
Palm oil fronds | 25.0 | 25.0 | 25.0 | 23.0 | 23.0 | |||
Cassava waste | 23.0 | 20.0 | 18.0 | 22.0 | 20.0 | |||
Tofu waste | 17.0 | 15.0 | 15.0 | 15.0 | 15.0 | |||
Sugarcane molasses | 20.0 | 20.0 | 20.0 | 20.0 | 20.0 | |||
Urea | 1.5 | 1.5 | 1.5 | 1.5 | 1.5 | |||
Premix* | 1.5 | 1.5 | 1.5 | 1.5 | 1.5 | |||
L. plantarum | 0 | 1.5 | 1.5 | 1.5 | 1.5 | |||
S. cerevisiae | 0 | 1.5 | 1.5 | 1.5 | 1.5 | |||
P. aeruginosa | 0 | 1.0 | 2.0 | 1.0 | 2.0 | |||
A. baumannii | 0 | 1.0 | 2.0 | 1.0 | 2.0 |
*Premix provided the following per kilogram diet: 270 g calcium, 189 g phosporus, 12 g magnese, 300.000 IU Vitamin A, 50.000 IU Vitamin D3, 100 mg Vitamin E, 100 mg Vitamin K, 20 g trace element (Zn, Mn, Fe, Cu, I, Co, Mo, Se).
Table 2: The chemical compositions of the complete feed block
Variables | Experimental Feed Blocks | SEM | P-value | |||||
A | B | C | D | E | ||||
Dry matter (%) | 80.9 | 81.4 | 80.9 | 80.5 | 80.2 | 0.58 | 0.69 | |
Organic matter (%) | 90.6 | 90.1 | 89.6 | 90.1 | 89.8 | 0.46 | 0.76 | |
Crude protein (%) |
10.5b |
11.0ab |
12.5a |
12.3a |
11.9a |
0.17 | 0.01 | |
NDF (%) | 48.3 | 46.9 | 46.4 | 47.3 | 46.4 | 0.17 | 0.55 | |
ADF (%) |
31.3a |
29.3b |
28.7b |
29.2b |
28.9b |
0.55 |
0.04 |
|
L. plantarum (cfu/g) |
- |
8×105 |
6×105 |
2.2×106 |
3.5 ×106 |
|||
S. cerevisiae (cfu/g) |
- |
9×105 |
4×105 |
4×105 |
2,1×108 |
|||
P. aeruginosa (cfu/g) |
- |
1×105 |
1×105 |
5×105 |
2×105 |
|||
A. baumanni (cfu/g) |
- |
3×105 |
1×105 |
8×107 |
4×105 |
SEM: standard error of means; A: complete feed block without microbe; B: complete feed block containing 1.5% L. plantarum, 1.5% S. cerevisiae and 1% P. aeruginosa; C: complete feed block containing 1.5% L. plantarum, 1.5% S. cerevisiae, 2% P. aeruginosa and 2% A. baumanni; D: complete feed block containing 1.5% L. plantarum, 1.5% S. cerevisiae, 1% P. aeruginosa and 1% A. baumanni; E: complete feed block containing 1.5% L. plantarum, 1.5% S. cerevisiae, 2% P. aeruginosa and 2% A. baumanni
Means with different superscripts in the same row differ significantly (P<0.05) or (P<0.01)
At the end of the incubation period, approximatelly 10 ml of syringe contents were sampled. The pH of medium was immediately recorded using a pH digital meter (Hanna, Hi 8520, Ronchi di Villafranca, Italy). Subsequently, 0.2 ml of sub-samples were pipetted into 1.5 ml micro centrifuge tube containing 1 ml of 25 g/100 ml (w/v) metaphosphoric acid and centrifuged at 9000 × g for 10 min for volatile fatty acids (VFA) determination. For NH3-N analysis, an additional 2 ml of sub-samples were added to 2 ml of 20 g/l (w/v) NaCl.
In vitro Nutrients Digestibility
Determinations of DM, organic matter (OM) and neutral detergent fiber (NDF) digestibility were conducted using modification in vitro procedure of Tilley and Terry (1963) as previously demonstrated by Santoso et al. (2016); Santoso et al. (2017). A total 25 ml of rumen liquor-buffer mixtures in a 1 : 4 (v/v) ratio were dispensed in 100 ml glass tubes that contained 250 mg dry sample. Triplicates of blank (with no feed sample) and standard (Pangola grass) samples were included in each run. Rumen liquor was collected in the morning prior to feeding and strained through four layers of cheese cloth into a pre-warmed thermos flask. After gassing CO2 in the tube, corks were tightly placed over the tubes and were incubated in a water bath at 39°C for 48 h. After 48 h of microbial incubation, samples were incubated at 39°C for 48 h with acid-pepsin. Therefore, the contents were filtered through pre-weighed Gooch crucibles and dried at 105°C for 24 h. The percent loss in weight was determined and presented as in vitro DM digestibility (IVDMD) and in vitro NDF digestibility (IVNDFD). The remaining residue was ashed at 550°C to determine in vitro OM digestibility (IVOMD).
Chemical Composition Determination
Samples of the feed were dried at 60 °C for 72 h in a forced-air oven, ground through a 1mm mesh sieve and analyzed for DM according to procedure of AOAC International (2005). The ash concentratios were determined after 2 h of oxidation at 600°C in a muffle furnace. Nitrogen content was measured by the Kjeldahl method. The fiber content i.e. NDF and acid detergent fiber (ADF) were which form the cell wall components in the samples, were analysed according to the methods reported by Van Soest et al. (1991). The NDF was determined without the use of μ-amylase and sodium sulfite, and the value was expressed inclusive of residual ash.
Statistical Analysis
Data were analyzed as a completely randomized design with 5 treatments and 3 replicates of treatments using the GLM procedure of SAS (version 9.1; SAS Institute, Cary, NC, USA). A comparison of means by Duncan’s multiple range tests was carried out at the probability of 5% and 1% levels.
Results and discussion
Chemical Composition of Complete Feed Block
The chemical composition of the complete feed blocks are presented in Table 2. Crude protein and ADF contents was significantly affected by treatments (P<0.01) and (P<0.05), respectively. The CP content was higher in feed block C, D and E than in feed block A (control). The ADF content were lower (P<0.05) in feed block with addition of mixed microbe (B, C, D, E) than in feed block A. The NDF and ADF contents in feed block containing 2% P. aeruginosa and A. baumannii (C and E) were slightly lower than feed block containing 1% P. aeruginosa and A. baumannii. The lower NDF and ADF content might be partly caused by present of cellulolytic bacteria in the feed block. Oyeleke and Okusanmi (2008) reported that P. aeruginosa was found in the rumen of cow, sheep and goat and was able to hydrolyse cellulose. Chang et al. (2015) noted that Acinetobacter sp. was found in the rumen of Korean native cattle and it was belonged to cellulolytic bacteria. Furthermore, Van Soest (1994) stated that microbial enzymes can reduce the levels of NDF.
The average DM and OM contents in all complete feed blocks were 80.8% and 90.0%, repectively which were similar to complete feed block based on palm oil fronds as previously reported by Santoso et al. (2017). The average CP content was 11.6%, which is above the threshold value of 7%. Minson and Milford (1966) noted that the digestibility declines when the animals are fed herbage with a CP content below 7% because microbial activity in the rumen becomes depressed by the lack of nitrogen.
The population of microbes in the complete feed block were 105-106 cfu/g of L. plantarum, 105-108 cfu/g of S. cerevisiae, 105 cfu/g of P. aeruginosa, and 105-107 cfu/g of A. baumanni. The population of L. plantarum, S. cerevisiae and A. baumanni in the complete feed block was slightly higher, otherwise the population P. aeruginosa was slightly lower than complete feed block as previously reported by Santoso et al. (2017).
In vitro Rumen Fermentation Characteristics
The pH value, concentrations of NH3-N, individual and total VFA are shown in Table 3.The pH value in feed block D and E was higher (P<0.01) than feed block A, B and C. In contrast, Sheikh et al. (2017) conluded that additions of probiotic in feed complete reduced in vitro rumen pH. However, the pH value in all treatments was within the range considered optimal for microbial digestion activity. Olson (1997) concluded that the fiber-digesting bacteria thriving best at pH 8.0 to 6.8 and starch-digesting bacteria at 5.5 to 6.0, the best balance of fiber and starch digestion occurs at a rumen pH of around 6.0. Meanwhile, the optimal ruminal pH is from 5.8 to 6.0 for fiber digestion (Kolver and De Veth, 2002).
In the rumen protein and other nitrogenous compounds are broken down into ammonia and peptides. The ammonia is used by the microbes for their protein synthesis.Concentration of NH3-N was increased (P<0.01) by supplementation with 2% P. aeruginosa and A. baumanni (C and E), which was attributed to enhanced dietary protein degradation by increased proteolytic bacterial population. The optimal concentration of rumen NH3-N required to maximize microbial microbial protein syntesis is 8.5 to over 30 mg dl-1 (McDonald et al., 2010). In other study, Abdulrazak et al. (1997) noted that 5-8 mg 100 ml-1 rumen liquor could be sufficient for fiber digestion. Thus, in present study, the ammonia N in all treatments of feed block was sufficient to ensure optimum microbial growth and fiber digestion.
The addition of 2% cellulolytic bacteria in feed block C and E resulted in higher (P<0.01) production of total VFA than the feed block with 1% cellulolytic bacteria (B and D). Generally, as cellulose and hemicellulose levels increase, relative to the amounts of soluble carbohydrate and starch levels, the acetate: propionate ratio also increases as a function of both increased acetate and decreased propionate (Murphy et al., 1982). Moreover, Djikstra (1994) concluded that fermentation of structural carbohydrates, compared to fermentation of starch, yield high amounts of acetic and low amounts of propionic acid.
As a known LAB, L. plantarum is expected to increase lactic acid production in feed block B, C, D and E which may stimulate the growth of lactate utilizing microorganisms,
Table 3: In vitro fermentation characteristics in the supernatant after 48 h of incubation
Variables | Experimental Feed Blocks | SEM | P-value | |||||
|
A | B | C | D | E |
|
|
|
pH |
6.67a |
6.82ab |
6.83ab |
6.86a |
6.92a |
0.04 |
0.01 |
|
NH3-N (mg/100 ml) |
33.1b |
34.7b |
41.0a |
34.6b |
39.5a |
0.44 |
0.01 |
|
Acetic acid (A) (mM) |
17.5b |
19.4a |
20.4a |
19.2a |
19.5a |
0.46 |
0.05 |
|
Propionic acid (P) (mM) |
11.8c |
13.3bc |
13.4ab |
12.4bc |
13.7a |
0.27 |
0.01 |
|
Butyric acid (mM) |
10.9ab |
10.4b |
10.2b |
11.6a |
11.8a |
0.25 |
0.01 |
|
A : P |
1.48 |
1.46 |
1.52 |
1.55 |
1.42 |
0.03 |
0.09 |
|
Total VFA (mM) |
40.2b |
43.1ab |
44.0a |
43.3ab |
45.0a |
0.71 |
0.01 |
|
Total gas 48 h (ml/300 mg) |
45.8 |
46.3 |
49.1 |
49.6 |
51.0 |
1.64 |
0.19 |
|
CH4 (ml/300 mg) |
8.34a |
8.26ab |
6.99b |
5.20c |
5.20c |
0.28 |
0.01 |
|
SEM: standard error of means; A: complete feed block without microbe; B: complete feed block containing 1.5% L. plantarum, 1.5% S. cerevisiae and 1% P. aeruginosa; C: complete feed block containing 1.5% L. plantarum, 1.5% S. cerevisiae, 2% P. aeruginosa and 2% A. baumanni; D: complete feed block containing 1.5% L. plantarum, 1.5% S. cerevisiae, 1% P. aeruginosa and 1% A. baumanni; E: complete feed block containing 1.5% L. plantarum, 1.5% S. cerevisiae, 2% P. aeruginosa and 2% A. baumanni
Means with different superscripts in the same row differ significantly (P<0.05) or (P<0.01)
Table 4: In vitro digestibility of dry matter, organic matter and neutral detergent fiber of the complete feed block
Variables | Experimental Feed Blocks | SEM | P-value | ||||
A | B | C | D | E | |||
IVDMD (%) | 53.8 | 55.6 | 56.6 | 55.6 | 56.6 | 0.82 | 0.19 |
IVOMD (%) |
61.8c |
63.7abc |
64.6ab |
63.3bc |
65.4a |
0.57 | 0.05 |
IVNDFD (%) |
32.4d |
35.2c |
38.6a |
36.2bc |
38.2ab |
0.49 |
0.01 |
SEM: standard error of means; A: complete feed block without microbe; B: complete feed block containing 1.5% L. plantarum, 1.5% S. cerevisiae and 1% P. aeruginosa; C: complete feed block containing 1.5% L. plantarum, 1.5% S. cerevisiae, 2% P. aeruginosa and 2% A. baumanni; D: complete feed block containing 1.5% L. plantarum, 1.5% S. cerevisiae, 1% P. aeruginosa and 1% A. baumanni; E: complete feed block containing 1.5% L. plantarum, 1.5% S. cerevisiae, 2% P. aeruginosa and 2% A. baumanni
Means with different superscripts in the same row differ significantly (P<0.05) or (P<0.01)
leading to the production of propionic acid. This finding is consistent with Soriano et al. (2014) who reported that addition of Lactobacillus mucosae in substrate of brewers’ grain increased the in vitro concentration of propionic acid.
Gas production can be used as an indicator of feed degradation in the rumen. Beuvink and Spoelstra (1992) noted that there was a significant correlation beetwen OM digestibility, VFA concentrations and gas production. Table 3 shows that total gas production during a 48 h incubation was not affected by combination of LAB, yeast and cellulolytic bacteria. However, addition of LAB, yeast and cellulolytic bacteria tended to increase gas production. These results are consistant with Tang et al. (2008) who reported an increased rate of gas production and cumulative gas volume for cereal straws by addition of yeast culture. Yeast supplementation to low quality basal forages promoted the growth of fibrolytic bacteria through its ability to scavenge oxygen and production of metabolites such as peptides, amino acids and branched-chain organic acids in the rumen (Fonty et al., 2006). Besharati, (2015) concluded that addition of S. cerevisiae at 2.5 g/kg DM in biscuit waste improved in vitro gas production parameters. The ability of yeast to increase in vitro gas production has been also reported by various authors with different roughages (Chaucheyras-Durand et al., 2008). The pattern on in vitro gas production of complete feed block enriched by mixed microbes at different incubation times are shown in Figure 1.
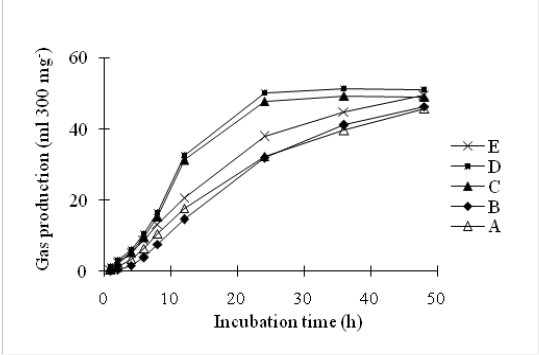
Figure 1: The pattern on in vitro gas production affected by addition of mixed microbes in complete feed block at different incubation times
Methane production from cattle in the tropics averaged 10–11% of gross energy intake. Methane production reduced (P<0.01) by 16.2%, 37.6% and 37.6% for feed block C, D and E, respectively when compared to feed block A. It has been demonstrated that CH4 production was suppressed by addition of S. cerevisiae (Lila et al., 2004) and L. plantarum (Santoso et al., 2016). The decrease CH4 concentration observed in concentrate containing LAB may due to the utilization of metabolic hydrogen by propioni bacteria to produce propionic acid. Ruminal propionic acid production competes with methanogenesis for available H2, thus increased propionic acid formation is stoichiometrically associated with decreased CH4 production, resulting in a decreased acetic acid to propionic acid ratio in the rumen (Seo et al., 2010).
Dry Matter, Organic Matter and Neutral Detergent Fiber Digestibility
Addition of 2% of P. aeruginosa and A. baumanni (C and E) in complete feed block had higher OM digetibility (P<0.05) and NDF digestibility (P<0.01) compared to control feed (A) (Table 4). The higher digestibility of NDF could be due to addition of cellulolytic bacteria into the feed block. Oyeleke and Okusanmi (2008) revealed that P. aeruginosa was found in the rumen of cow, sheep and cow approximately 9% of the bacteria species and was able to hydrolyse cellulose. This result is consistant with previous study by Santoso et al. (2017) reported addition of 1% of P. aeruginosa and A. baumanni in feed block had the highest in vitro digestibility of dry matter, organic matter and neutral detergent fiber as compared to other feed. Zain et al. (2015) concluded that the addition of some microbes as probiotics in feed could stimulate the microbes of the rumen and improve the digestibility of feed in ruminant livestock. Moreover, Chaucheyras et al. (1995) noted that S. cerevisae showed an ability to provide growth factors, such as organic acids or vitamins, thereby stimulating ruminal populations of cellulolytic bacteria. The present in vitro results should be tested further using in vivo experiments to evaluate the effects of combination lactic acid bacteria, yeast and cellulolytic bacteria on performance in ruminants in the future.
Conclusions
This study demonstrated that addition of LAB, yeast and cellulolytic bacteria in complete feed block increased crude protein and reduced ADF contents. The complete feed block containing LAB, yeast and cellulolytic bacteria was effective in modifying ruminal fermentation patterns by increasing concentrations of NH3-N, total VFAs, and decreasing CH4 production. Additionally, digestibility of OM and NDF in feed block added mixed microbes were higher than those of control feed block in in vitro fermentation.
Acknowledgments
This study was funded by The Directorate General of Strengthening Research and Development, Indonesian Ministry of Research, Technology and Higher Education (contract number 089/SP2H/LT/DRPM/IV/2017). The authors are grateful to A. I. Mustakim and Y. Supriantifor the technical assistance to prepare the sample.
Conflict of Interest
The authors declare that they have no conflict of interests.
AUTHORS CONTRIBUTION
All the authors contributed significantly to the paper.
References