Advances in Animal and Veterinary Sciences
Research Article
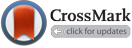
Development of Oocytes and Preimplantation Embryos of Mice Fed Diet Supplemented with Dunaliella salina
Abd El-Nasser Ahmed Mohammed1,2
1Department of Animal and Fish Production, College of Agriculture and Food Sciences, King Faisal University, Saudi Arabia; 2Department of Animal Production, Faculty of Agriculture, Assiut University, Egypt, 71526.
Abstract | The present study investigated the developmental competence of germinal vesicle (GV) oocytes and the resulting embryos upon dietary Dunaliella salina (DS) supplementation. Quality of GV oocytes and maturation, timing of zygotes cleavage to two-cell stage, quality of embryos and offspring number and weight were investigated of mice supplemented with DS (100g/kg ration; N=30) compared to control (N=30).Females were injected with 7.5 I.U. of equine chorionic gonadotropin (PMSG) followed by 7.5 I.U. of human chorionic gonadotropin (hCG) after 48h and mated with fertile male. Immature GV oocytes were harvested from ovaries after 48h of PMSG injection for investigating quality of oocyte, timing of breakdown of germinal vesicle (GVBD) and extrusion of first polar bodies and maturation rate (%).Zygotes were harvested from uterine tubes 29-30hafterhCG injection and followed for first cleavage whereas quality of blastocysts were evaluated after collection from uterine horn at96h of hCG. Improvement of oocyte quality has been indicated in DS group whereas GVBD and maturation rate were not differed between DS and control groups. Although D. salina did not change timing of first cleavage of zygotes, blastocysts’ quality were significantly (P<0.05) increased in DS group. Furthermore, offspring number (9.9 ± 0.26 & 8.2 ± 0.30) and weight (12.01 ± 0.31 & 9.84 ± 0.37) at birth were increased significantly (P<0.05) in the DS group compared to control one. In conclusion, supplementation of D. salina could increase reproductive performance through improvement quality of germinal vesicle oocytes and preimplantation embryos.
Keywords | Microalgae; Dunaliella salina; Oocytes, Embryos; Reproductive performances
Editor | Kuldeep Dhama, Indian Veterinary Research Institute, Uttar Pradesh, India.
Received | July 04, 2017; Accepted | December 25, 2017; Published | December 28, 2017
*Correspondence | Abd El-Nasser Ahmed Mohammed, Department of Animal and Fish Production, College of Agriculture and Food Sciences, King Faisal University, Saudi Arabia; Email: aamohammed@kfu.edu.sa
Citation | Mohammed AA (2018). Development of oocytes and preimplantation embryos of mice fed diet supplemented with dunaliella salina. Adv. Anim. Vet. Sci. 6(1): 33-39.
DOI | http://dx.doi.org/10.17582/journal.aavs/2018/6.1.33.39
ISSN (Online) | 2307-8316; ISSN (Print) | 2309-3331
Copyright © 2018 Mohammed et al. This is an open access article distributed under the Creative Commons Attribution License, which permits unrestricted use, distribution, and reproduction in any medium, provided the original work is properly cited.
INTRODUCTION
Recent interest has grown for the use of green microalgae including Dunaliella salina (D. salina) as a novel feed supplement for nutritional purposes (Yaakob et al., 2014) or detoxification and chelating of toxic and heavy metals. D. salina grant various health effects to the humans and animals as well. D. salina can protect against CNS oxygen toxicity (Bitterman et al., 1994) and gastrointestinal inflammation (Lavy et al., 2003) through anticancer and antioxidant properties because of its content of high β-carotene (Krinsky, 1988). Moreover, D. salina has immunomodulatory and anti-inflammatory effects (Hemmingson et al., 2006; Abdel-Daim et al., 2015). D. salina improved body growth and reproduction (Nagasawa et al., 1989).Supplementation of algae or algal oil to dairy cattle increase n-3 percentage in milk fat without adverse effect on milk fat content (Stamey et al., 2012).
Stimulation of ovarian activity was widely explored through nutrition (Downing et al., 1995; Gifre et al., 2017), hormonal administration (Mandiki et al., 1993; Zarazaga et al., 1996), both nutritional and hormonal supplementation (Mohammed and Attaai, 2011). Because of the negative impacts of increased protein and energy in the diet on health and fertility (McEvoy et al., 1997; Dawuda et al., 2002) and the high cost of ration, it is important to search for non-traditional supplements with beneficial characteristics for body. Microalgae possesses high concentration of proteins, lipids and vitamins, sulfated polysaccharides, several amounts of polyunsaturated fatty acids (Gouveia et al., 2008; Ghasemi et al., 2011; Wichuk et al., 2014; Cuellar-Bermudez et al., 2015; Gong and Bassi; 2016). Pulz and Gross (2004) showed that polysaccharides is important as therapeutic where it support immune system and has potential role to fight virus infection (Abdel-Daim et al., 2015). Several studies has been indicated that protein content in most of green microalgae is higher than plants (Becker, 1994; Becker, 2007). Microalgae consequently gift to humans and animals positive healthy and reproductive effects (Becker, 2007; Guedes et al., 2013; Choochote et al., 2014; Senosy et al., 2017).
The stimulatory effects of dietary green microalgaeon reproductive performance has been confirmed. Conclusion of our work (Senosy et al., 2017) indicated that D. salina supplemented to Boer goats (10 g/day/head) accelerated ovarian follicle development and significantly increased follicle numbers (small, medium and large follicles). In addition, plasma metabolites and hormones (FSH, LH, estrogen and progesterone) were significantly increased upon supplementation of D. salina. Therefore, this study was conducted to explore beyond such information as development of oocytes and embryos. Kinetics of oocytes and embryos cleavages indicated differences either in vitro or in vivo (Mohammed et al., 2005; Mohammed and Attaai, 2011). First cleave of embryos and development was changed upon maturation of bovine oocytes in the presence of follicular fluid (FF) or fetal calf serum (FCS) (Mohammed et al., 2005). Because of the beneficial effects of D. salina on reproduction, the present work carried out to explore such improvement through the development of oocytes and embryos. Therefore, the objectives of the present research were to explore kinetics of oocyte and embryo cleavages in addition to reproductive performances upon feeding D. salina in mice.
MATERIALS AND METHODS
All organic and inorganic materials used in this work were obtained from Caisson Lab (USA), unless stated otherwise. All media were prepared fresh and sterilized through 0.2- µm syringe filter (Nalgene, Cat. No. 190-2520).
Site of Study and Management
The study was conducted according to procedures approved by the Ethics Committee of Animal Experimentation of King Faisal University, Saudi Arabi. The study was conducted during the period from March to May 2017. Mice were kept controlled under cycle of 12h light and 12h dark. The controlled temperature and relative humidity during the experiment were 25 ±3ºC and 50 ±10%, respectively. Mice are fed commercial pellets of basal control diet (Arasco, KSA) or basal control diet supplemented with D. salina (100g/kg diet) and had free access to food and water for at least 4 wk.
Animals and Feeding
Sixty female albino mice of age 6-8 weeks were used during the study. The female were classified into two groups; the control group fed basal diet obtained from Arasco (KSA), which composed of 21.0%, 2.9% fat and 3.3% fiber,1% mixture of vitamins and minerals (Table 1) and 3300 kcal/kg energy. In addition, D. salina algae added to basal diet (100 g/kg). D.salina microalgae were produced by Aquaculture National Group (KSA). The chemical composition of the experimental D. salina algae using standard methods (AOAC, 1990) was 8.47% ± 0.12moisture,54.17% ± 0.47crude protein, 0.80% ± 0.10 fiber, 11.42% ± 0.22 total lipid, 18.47% ± 0.26 ether extract and6.67% ± 0.23 ash.
Table 1: The mixture of vitamins and minerals added to the basal control diet
Vitamins mixture | Vitamins mixture | ||
Vitamin A | 2000000, IU/kg | Folic acid | 800, mg/kg |
Vitamin D | 300000, IU/kg | Pantothenic acid | 5000, mg/kg |
Vitamin E | 40000, IU/kg | Biotin | 200, mg/kg |
Vitamin C | 60000, mg/kg | Antioxidant | 20000, mg/kg |
Vitamin K | 1200, mg/kg | Cobalt | 200, mg/kg |
Vitamin B1 | 4000, mg/kg | Copper | 1000, mg/kg |
Vitamin B2 | 4000, mg/kg | Iron | 4000, mg/kg |
Vitamin B6 | 2400, mg/kg | Manganese | 15000, mg/kg |
Vitamin B12 | 10, mg/kg | Selenium | 20, mg/kg |
Niacin | 24000, mg/kg | Zinc |
15000, mg/kg |
Collections of Germinal Vesicle Oocytes
Females were given 7.5 I.U. of pregnant serum gonadotropin (PMSG, Intervet). Female mice were killed through cervical dislocation 44-48 h after PMSG injection. Ovaries were removed from the donor females. The GV-stage oocytes of ovarian follicles were released by puncturing with 30G sterile needles under a stereomicroscope. The cumulus-enclosed GV oocytes were released into HEPES tissue cell culture medium (TCM 199) supplemented with 5% fetal bovine serum. The cumulus-enclosed GV oocytes were immediately collected using glass pipette, its tip diameter larger than the diameter of cumulus-enclosed GV oocytes. The collected oocytes were graded into cumulus-enclosed GV oocytes (good oocytes), partial cumulus-enclosed GV oocytes (fair oocytes) and denuded oocytes (Figure 1A, B, C) (Mohammed and Attaai, 2011).
Timing of Germinal Vesicle Breakdown (Gvbd) and Extrusion of First Polar Body
Cumulus cells were removed from oocytes using glass pipette, its tip diameter was smaller than cumulus-enclosed GV oocytes diameter. The denuded oocytes were cultured in TCM199 supplemented with 10.0% FCS. The oocytes were investigated for timing of GVBD and extrusion of first polar body during in vitro maturation (Figure 1D, E, F) (Mohammed et al., 2008; Mohammed et al., 2010; Mohammed, 2014).

Figure 1: Categorizing of developmental competence of oocytes and embryos upon D. salina supplementation in mice; A) whole cumulus-enclosed germinal vesicle (good) oocytes; B) partial cumulus-enclosed germinal vesicle (fair) oocytes; C) denuded (bad) oocytes ; D) germinal vesicle breakdown; E) processing of polar body extrusion stage; F) polar body extruded; G) late 1-cell stage embryo prior of cleavage; H) processing of first cleavage; I) two-cell stage embryo; J) Good quality embryo; K) fair quality embryo; L) bad quality embryo.
Collection of Cleaving Embryos
The females mice were super ovulated by 7.5 I.U. injection of equine chorionic gonadotropin (PMSG, Intervet) followed 48 h later by 7.5 I.U. injection of human chorionic gonadotropin (hCG, Intervet) and mated with fertile males. The same males were used to inseminate both females of control and DS group to exclude the paternal effect on embryo first cleavages. Late 1-cellstage embryos were collected in Dulbecco’s modified medium supplemented with 10% FCS from the oviducts 29-30 h after hCG injection. The collected late 1-cell stage embryos were washed and cultured in KSOM+AA medium in vitro and followed for cleavage every 15 min (Figure 1 G, H, I).
Quality of Collected Embryos
Embryos were flushed from the uteri at4.00 days post hCG injection by using Dulbecco’s modified medium supplemented with 10% FCS. The collected embryos graded into three categories; good, fair and bad embryos (Figure 1 J, K, L).
Reproductive Performance
Females were checked for parturition 4 times/day since day 18 of hCG injection. The numbers and weight of pops per female were counted and weighted for each group.
Statistical Analysis
Data are presented as mean ± SEM. The results were subjected to statistical analysis with independent t-test (SAS 2008). Comparison was done between control and D. salina treated groups. Values of probability of less than 0.05 (P < 0.05) were considered significant.
RESULTS
Oocyte Quality
The GV-stage oocytes of ovarian follicles collected 44-48 h after PMSG injection were graded into good fair and bad quality. Quality of oocytes was improved upon D. salina supplementation. Percentage of cumulus enclosed GV (good) oocytes were significantly (P < 0.05) increased whereas denuded (bad) oocytes were significantly decreased in D. salina group compared to control one. The percentage of good, fair and denuded oocytes in D. salina group was 71.6± 1.29% (43/60), 20.0± 0.85% (12/60) and 8.4± 0.43% (5/60), respectively versus50.0±1.29% (30/60),15.0± 0.43 (9/60)and 35.0 ±0.85 (21/60) in control one (Figure 2).
a,b; Values with different superscripts between D. salina and control groups significantly differed at P < 0.05.
Timing of Germinal Vesicle Breakdown (Gvbd) and Extrusion of First Polar Body
Sixty GV-stage oocytes cultured for in vitro maturation were investigated for timing of GVBD and extrusion of first polar body. No differences observed in timing of GVBD of D. salina and control oocytes, which occurred 2-3 hr. of starting maturation. Extrusion of 1stpolar bodies of both D. salina and control oocytes occurred 9-12 h (Figure 3). It seems as D. salina supplementation slightly accelerated timing of polar body extrusion and did not change (P > 0.05) percentage of oocyte maturation (96.66%± 0.74;58/60 vs. 95.0%± 1.29; 57/60).

Figure 3: Percentage of oocytes at timing extrusion of first polar body of D. salina and control oocytes.
Timing of Embryo Cleavage and Quality and Reproductive Performance
Sixty late 1-cell stage embryos collected at 29-30 hr. of hCG injection of both D. salina and control were explored for first cleavages. Timing explored for first cleavage of late 1-cell stage embryos to two-cell stage embryos was not differed between D. salina and control group where all cleavages occurred within one hour and half. One hundred and thirty embryos were collected from ten females of D. salina group whereas one hundred and thirteen were collected from ten females of control group at day 4.0 of hCG injection. Percentage of embryos of good, fair and bad quality were significantly differed between D. salina and control groups. Percentage of embryos of good quality (62.16% ± 1.09 vs. 54.77% ± 1.38) and fair quality (23.51% ± 2.46vs. 18.97% ± 2.40) were increased in D. salina group compared to control one. On the other hand, Percentage of embryos of bad quality were decreased in D. salina group compared to control one (14.30% ± 1.84 vs. 26.24%± 1.72) (Figure 4).
Furthermore, average of offspring number(9.9 ± 0.26 & 8.2 ± 0.30) and weight (12.01 ± 0.31 &9.84 ± 0.37)at birth of ten deliveries were increased significantly (P<0.05) in the D. salina group compared to control one (Figure 5).
a,b; Values with different superscripts between D. salina and control groups significantly differed at P < 0.05.

Figure 5: Offspring number and weight (g) of litter size of D. salina (100g/kg diet) and control groups.
a,b; Values with different superscripts between D. salina and control groups significantly differed at P < 0.05.
DISCUSSION
In the current study, the effects of D. salina supplementation on development of oocytes and embryos in addition to reproductive performance of mice were explored. D. salina, as an important type of green microalgae, supplementation improved oocyte and embryos development. Furthermore, reproductive performances including number and weight at birth were improved significantly (P < 0.05) upon D. salina supplementation. Nutritional supply, D. salina, may be used as an alternative to hormonal treatments to enhance reproductive performance. D. salina has high levels of beta-carotene, glycerol, protein and other fine chemicals (Gouveia et al., 2008; Ghasemi et al., 2011; Wichuk et al., 2014; Cuellar-Bermudez et al., 2015; Gong and Bassi, 2016).
Oocyte Quality
There are significant increases of cumulus-enclosed GV-oocytes (71.6% ± 1.29 vs. 50.0% ± 1.29) and partial cumulus-enclosed GV-oocytes (20.0% ± 0.85 vs. 15.0% ± 0.43), respectively in D. salina group compared to control one. D. salina has high levels of beta-carotene, glycerol, protein and other fine chemicals (Gouveia et al., 2008; Ghasemi et al., 2011; Wichuk et al., 2014; Cuellar-Bermudez et al., 2015; Gong and Bassi; 2016), which might improve the quality of oocyte and support further embryonic development. Conclusion of our work (Senosy et al., 2017) indicated that D. salina supplemented to Boer goats (10 g/day/head) accelerated ovarian follicle development and significantly, increased follicle numbers (small, medium and large follicles).The improvement of oocyte quality in D. salina group compared to control one might be related to high levels of beta-carotene and elevation of plasma metabolites and hormones levels. Short-term beta-carotene supplementation in goat affects positively on serum insulin concentrations and ovarian activity (Meza-Herrera et al., 2013). Several studies suggest a direct role for insulin action on female reproduction. Senosy et al. (2017) found that D. salina supplementation to Boer goats resulted in significant increase of plasma FSH hormone levels, which rescued cumulus cell apoptosis and enhanced developmental capacity of buffalo oocytes (Jain et al., 2016).
Timing of Germinal Vesicle Breakdown (Gvbd) and Extrusion of First Polar Body
No differences observed in timing of GVBD of D. salina and control oocytes, which occurred 2-3 hr. of starting maturation. Timing extrusion of 1st polar bodies of both D. salina and control oocytes occurred 9-12 hr. It seems as D. salina supplementation slightly accelerated timing of polar body extrusion and did not change percentage of oocyte maturation (96.66% ± 0.74 vs. 95.0% ± 1.29). Timing of GVBD and extrusion of first polar body affects arrangements of chromosomes during maturation and further embryonic development (Mohammed et al., 2008; Mohammed et al., 2010; Mohammed, 2014). It seems that the timing occurred of germinal vesicle breakdown and polar body extrusion supported further embryonic development and reproductive performance.
Timing of First Embryo Cleavage and Embryo Quality and Reproductive Performance
Although timing explored for first cleavage of late 1-cell stage embryos to two-cell stage embryos was not differed between D. salina and control group, percentage of embryos of good quality (62.16% ± 1.09 vs. 54.77% ± 1.38; P < 0.05) and fair quality (23.51% ± 2.46 vs. 18.97% ± 2.40; P > 0.05) were increased in D. salina group compared to control one. The improvement of developmental capacity of oocytes and embryos due to supplementation of female mice with D. salina (100g/kg diet) confirmed by the significant (P < 0.05) increase of number of litter size (9.9 ± 0.26 Vs. 8.2 ± 0.30; P < 0.023) and weight (12.02 ± 0.31 Vs. 9.84 ± 0.37; P < 0.023) at birth compared to control group. The improvement of number of litter size and weight might be attributed also to the beneficial effects of D. salina on body health and ovarian function. The recorded body weight during the experimental period indicates increasing of body weight gain by about 10.0% of D. salina group compared to control one as in other studies (Nagasawa et al., 1989).
High concentrations of essential fatty acids (EFAs) found in D. salina algae. These EFAs (Omega 3, Omega 6, linoleic acid) support function of normal reproduction and carotenoids absorption, vitamin E and other fat-soluble nutrients. There are beneficial effects of β-carotene of D. salina on reproduction reported of various species. β-carotene administration in mice (Chew and Arche, 1983) and pigs (Brief and Chew, 1985; Kostoglou et al., 2000) increased litter size and decreased embryonic mortality. Haliloglu et al. (2002) found a positive correlation between beta-carotene level and corpus luteum weight and function of pregnant cattle. In addition, dietary β-carotene supplementation in dairy cows decreased endometritis and retained placental (Michal et al., 1994). Schweigert et al. (2002) studied the effect of dietary β-carotene of gilts on uterine fluid composition and early embryonic development. They found that β-carotene resulted in a higher number of early developing embryos. β-carotene injections to super ovulated Holstein cows improved embryo quality (Sales et al., 2008).
There are significant increases upon D. salina supplementation of blood and plasma metabolites and hormones (FSH, LH, estrogen and progesterone) concentrations indicated in several studies (Alishahi et al., 2014; Senosy et al., 2017). Such improvement of follicles development in addition to the increase of plasma metabolites and hormones (Senosy et al., 2017), which positively effect on the subsequent quality of oocytes and embryos might be the reason of the significant (P < 0.05) increase of number and weight of litter size at birth in this study.
CONCLUSION
Supplementation of D. salina could increase reproductive performance of mice through the improvement of oocyte quality and preimplantation embryo development. Further in vitro studies are still required to investigate the developmental competence of oocytes and embryos upon D. salina supplementation.
ACKNOWLEDGMENTS
The authors thank the Deanship of Scientific Research (Grant number 160077)
CONFLICT OF INTEREST
No conflict of interest for author to declare.
Authors COntribution
All authors contributed equally.
REFERENCES