Advances in Animal and Veterinary Sciences
Research Article
Advances in Animal and Veterinary Sciences 2 (3): 177 – 182Use of Polymeric Scaffold for In Vitro Growth of Fibroblast–Like Cells of Indian Major Carp, Cirrhinus Mrigala
Pramod Kumar Nanda2, Priyabrat Swain1*, Sukanta Kumar Nayak1, Sudhansu Sekhar Mishra1, Pallipuram Jayasankar1, Sanjeeb Kumar Sahoo3
- Central Institute of Freshwater Aquaculture, Kausalyaganga, Bhubaneswar–751 002, India
- Indian Veterinary Research Institute, Eastern Regional Station, Belgachia-700 037, Kolkata, India
- Institute of Life Sciences, Bhubaneswar –751 023, India
*Corresponding author: pswainy2k@yahoo.co.in
ARTICLE CITATION:
Nanda PK, Swain P, Nayak SK, Mishra SS, Jayasankar P and Sahoo SK (2014). Use of polymeric scaffold for in vitro growth of fibroblast–like cells of Indian Major Carp, Cirrhinus mrigala. Adv. Anim. Vet. Sci. 2 (3): 177 – 182.
Received: 2014–01–26, Revised: 2014–02–25, Accepted: 2014–02–26
The electronic version of this article is the complete one and can be found online at
(
http://dx.doi.org/10.14737/journal.aavs/2014/2.3.177.182
)
which permits unrestricted use, distribution, and reproduction in any medium, provided the original work is properly cited
ABSTRACT
Cell culture has many applications in biological research. Now days, the focus has been to replace routinely practiced two–dimensional (2–D) cell culture with three–dimensional (3–D) cell culture systems due to their efficacy in increasing cell attachment, proliferation and differentiation. In this study, formulated poly lactic acid (PLA) scaffold blended with different natural polymers such as gelatin (PLA–G), alginate (PLA–A), dextran (PLA–D) and pectin (PLA–P), by multiple emulsion solvent evaporation method, were evaluated to find their effect on the attachment, growth and proliferation of fibroblast–like cells from heart of Indian Major Carp, Cirrhinus mrigala. Amongst the formulated scaffolds, PLA–A demonstrated significantly better growth of fibroblast–like cells as compared to control and other formulations. The effect of different seeding density (0.4, 0.6, 0.8, 1.2 and 1.6 × 10 5 cells ml–1 media) on cell proliferation and growth was also studied using PLA–A. PLA–A demonstrated more than 1.5 times higher cell proliferation and growth as compared to control (2–D) cell culture after 9th day post seeding, even with less cell seeding density (1.6 × 105 cells ml–1 media). However, with a seeding density of 0.8 × 10 5 cells ml –1 media, this scaffold formulation (PLA–A) supported best proliferation and growth of fibroblast cells. In short, PLA–A based hydrophilic scaffold formulation revealed its potential use in culture of anchorage dependent fibroblast–like cells from heart of Indian major carp, C. mrigala.
INTRODUCTION
In vitro culture of cells is traditionally done on two–dimensional (2–D) surfaces of tissue culture plastic. But in recent times, three–dimensional (3–D) scaffold systems are mostly preferred because of their wide application in biomedical and biological sciences. This is mainly due to the fact that 3–D culture systems using scaffolds can influence the physical, chemical and biological environment surrounding a cell population and their porous structures can be used to sustain cell adhesion/ attachment and proliferation (Voytik–Harbin, 2001; Yang et al., 2002; Zhu et al., 2002; Cui et al., 2003, Carletti et al., 2011). Further, 3–D cell culture mimics the in vivo microenvironment and increase cell–cell interactions compared to 2–D culture (Harrington et al., 2013). Several types of synthetic biodegradable polymers such as Poly–L–lactic–acid–biopolymer (PLLA), poly (lactic–co–glycolic) acid (PLGA), Poly–caprolactone (PCL), Polybutylene terephthalate (PBT) etc. are used as porous scaffolding materials in different systems. Amongst, poly–lactic acid (PLA), polyglycolide (PG), and their co–polymers are most widely used in the fabrication of polymeric scaffolds (Mikos et al., 1994a & b; Murphy et al., 2002; Sachlos and Czernuszka, 2003, Rasal et al., 2010) because of their biocompatibility and biodegradability (Mikos et al., 1993; Mooney et al., 1995; Mooney, 1999). However, the polymeric matrix composition and structures must be considered carefully when designing a 3–D cell culture system (Voytik–Harbin, 2001; Chung and King, 2011) so that they provide adequate mechanical strength and hydrophilicity.
Like its application in other fields of biological sciences, this technology also has tremendous potential in fishery science. This is mainly due to the requirement of short term and continuous cell cultures from economically important fish species for their application in various in vitro studies. Further, most of the primary organ cells are anchorage dependent and require specific environments that very often include the presence of a supporting material to act as a template for cell growth (Yang et al., 2002). But primary culture is mostly done in 2–D environments in which variable culture conditions and choice of methods, such as procedure for enzymatic digestion and coating of culture vessels, often affect the quality of the primary culture (Garrick, 2000; Mothersill et al., 1995) resulting in low proliferation and growth of cells in fish model. Thus, frequent preparation of cell cultures is necessary, if primary cells are routinely used for experimental purposes. As a result of the inadequacy of 2–D cell culture, there is a growing interest in culturing adherent eukaryotic cells in 3–D culture. 3–D cultures within a polymeric matrix (also termed scaffold) are proposed as a better model to study complex biological processes than cells grown monolayer (Abbott, 2003), as they mimic properly the in vivo tissue state.
As scaffolds provide the structural support for cell attachment and subsequent proliferation beyond any doubt and have certainly huge scope in fish cell culture practices, the hypothesis of our current experiment is to use polymeric scaffolds for evaluating their efficacy in terms of adhesion, growth and proliferation of fish cell. In this regard, we have formulated and screened PLA scaffold blended with four different natural polymers such as alginate (PLA–A), dextran (PLA–D), gelatin (PLA–G) and pectin (PLA–P) to find out a suitable polymeric scaffold by evaluating the adhesion, attachment, growth and proliferation of anchorage dependent fibroblast–like cells from heart of Indian Major Carp, Cirrhinus mrigala.
MATERIALS AND METHODS
Materials Used
PLA with intrinsic viscosity 0.17 dL/g was purchased from Birmingham Polymers, Inc. (Birmingham, AL). Poly vinyl alcohol (PVA) (average MW 30,000–70,000 Da), bovine serum albumin (BSA, Fraction V), sucrose, chitosan from crab shells (85% deacetylated), alginate, dextran, gelatin and pectin were purchased from Sigma Aldrich (St. Louis, MO, USA).
Formulation of Scaffold
Natural polymer blended PLA scaffolds (PLA–A, PLA–D, PLA–G and PLA–P) were prepared by the multiple solvent evaporation method with slight modifications (Sahoo et al., 2005). In brief, 800 l of aqueous phase (W1) was emulsified into the polymer solution (O) (200 mg of PLA polymer in 4 ml of dichloromethane) using a homogenizer (Biospacte Product Inc, Bartlesville, OK) at 121 × g to form a primary (W1/ O) emulsion. The internal aqueous phase (W1) contained 2.5 % w/v BSA, sucrose (10 % w/v), chitosan solution (1.25 % in 0.01 % v/v lactic acid), PVA (5 % w/v) and any one of the natural polymer from alginate, dextran, gelatin and pectin (5 % w/v). BSA was added into the internal aqueous phase to enhance stability of the primary emulsion. The primary emulsion was added drop wise into a 1 % w/v aqueous solution of PVA containing 10 % w/v sucrose (W2) under constant magnetic stirring on a stir plate to form a multiple emulsion (W1/O/W2). The emulsion was stirred overnight on a magnetic stir plate to evaporate the organic solvent. Scaffolds were recovered by centrifugation at 27,377 × g, washed three times with distilled water, and then lyophilized for 48 h (LABCONCO Corporation, USA) to get the lyophilized powder for further study.
Characterization of Scaffold
The shape and surface morphology of scaffolds were characterized by scanning electron microscopy (SEM). The powdered scaffolds were stuck on to a brass stub through double adhesive tape and gold–coated using a sputter gold coater at 20 KV (Hitachi, E–1010, Ion Sputter). The stub was fixed into the sample holder and placed in vacuum chamber of SEM (Hitachi S–3400N, USA) and observed under low vacuum. The average particle diameter was determined from the SEM pictures of different formulations of scaffold. To calculate the mean particle diameter, 50 particles were taken for measurement.
Swelling Property
The swelling properties of the different formulated scaffolds were determined by incubating them in phosphate buffered saline (PBS, pH 7.2) at room temperature. A known weight (Wo) of the individual scaffold formulation (in triplicate) was placed in 5 ml PBS for 5 h. The wet weight (Wt) of the scaffolds was determined immediately after the surface–adsorbed water was removed by filter papers.
The PBS uptake ratio of the individual scaffold formulations (Pa) was calculated as per the following formula–
Pa= (Wt–Wo) / Wo
Primary Culture of Heart Explants of Cirrhinus mrigala
Primary culture was obtained from explants of the heart tissue of C. mrigala fingerlings following the method described by Nanda et al. (2009). Briefly, the heart tissue of C. mrigala fingerlings (45 ± 5.0 gm) was excised aseptically and immersed immediately in Dulbecco's Modified Eagle's Medium (DMEM). Prior to cultivation, the tissues were minced to a size of approximately 1–2 mm3 and repeatedly washed with DMEM. Explants of heart tissues were distributed over 25 mm2 flasks pre–coated with 0.5 ml gelatin (2 % w/v) and kept for 3 h under semi–dried condition. After incubation, the flasks were fed with 5 ml of DMEM growth medium supplemented with glutamine (0.3 % w/v), non–essential amino acids solution (100 X), 10% fetal bovine serum (FBS) and antibiotic–antimycotic solution (Sigma–Aldrich, St. Louis, MO, USA) and incubated at 26 ± 1ºC. The flasks were routinely observed for growth, proliferation and formation of monolayer under phase–contrast microscope (Olympus, Japan). The fibroblast–like cells from the explants started proliferating within 3–4 d and reached confluency (80–90 %) after 12–14 d. Sub–culture was done as per the method described by Wolf and Quimby (1976) using non–enzymatic cell dissociation solution (Sigma, USA) and the total viable cells were counted by trypan blue dye exclusion method.
Effect of Various PLA Scaffold Formulations on Growth of Fibroblast–Like Cells
The following protocol was used to determine the suitability of various scaffold formulations on adhesion and growth of fibroblast–like cells from heart tissue of C. mrigala. Individual scaffold formulation was separately soaked in 70% alcohol (2 mg ml–1) for overnight at 4 0C and centrifuged at 12,167 × g for 10 min at 4 0C. Supernatant from each sample was discarded and different scaffold formulations, each in triplicate, were aseptically transferred to six well plates using 1 ml of DMEM. Plates without having any scaffold formulations were taken as control (2–D) culture. Fibroblast–like cells obtained from sub–culture of heart tissue was added in each well at a seeding density of 2.0 × 105 cells ml–1 media supplemented with FBS and antibiotic–antimycotic solution as described previously. Plates were incubated at 26 ± 10C replacing medium on every alternate day. The effect of various scaffold formulations on cell growth kinetics was observed over one week.
Based on the preliminary screening of different scaffold formulations, the best formulation (PLA–A) was processed further to evaluate the effect of different seeding density on growth and proliferation of fibroblast–like cells from heart of C. mrigala.
Effect of PLA–A on the Growth and Proliferation of Fibroblast–Like Cells at Varying Seeding Density
The effect of PLA–A on the growth and proliferation of fibroblast–like cells from heart of C. mrigala at varying seeding density (0.2 × 10 5 to 1.6 × 105 cells ml–1 media) was studied. Cells were seeded on to six well plates and added with constant amount of PLA–A (2 mg ml–1 media) and the growth kinetics was studied up to 9th day post seeding. Similarly, normal 2–D culture study was also conducted by seeding fibroblast–like cells from heart of C. mrigala @ 2.0 × 105 cells ml–1 media in six well plates
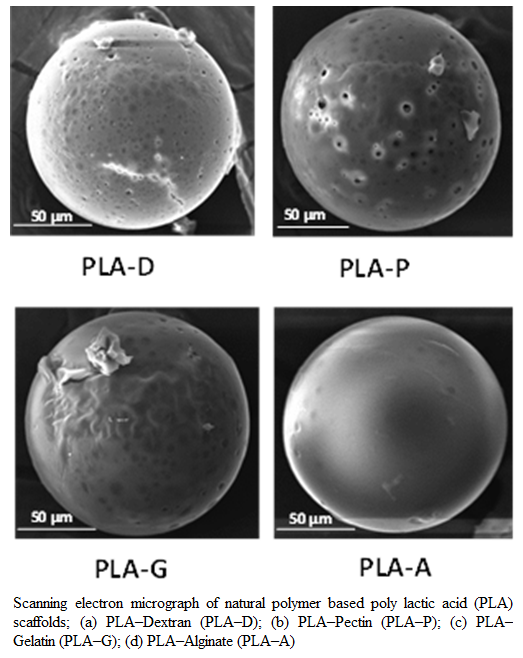
Figure 1:Scanning electron micrograph of natural polymer based poly lactic acid (PLA) scaffolds; (a) PLA–Dextran (PLA–D); (b) PLA–Pectin (PLA–P); (c) PLA–Gelatin (PLA–G); (d) PLA–Alginate (PLA–A)
PBS Swelling Behavior of Scaffold
without addition of scaffolds to compare the growth. Scaffolds were aspirated from the culture plates at different time points (3rd, 5th, 7th and 9th day) to determine the cell growth kinetics of proliferating cells. Photomicrographs of selected scaffold adhering cells were taken with an inverted microscope (Olympus, Japan). The total number of cells in all the scaffold clumps was determined following the method
described by Dhiman et al. (2004). Briefly, the cells from individual scaffold clump was first detached by treating to cell adhering scaffolds with 1 ml of 0.1 M citrate buffer
RESULTS
Formulation and Characterization of PLA Scaffold
In this study, four different natural polymer blended PLA scaffolds such as PLA–A, PLA–D, PLA–G and PLA–P were prepared by using multiple emulsion solvent evaporation method. In all the formulations of scaffolds, PVA was used in the external aqueous phase (W2) as an emulsion stabilizing agent and also, it was used in the internal aqueous phase (W1) of the primary emulsion. The overall objective was to formulate scaffold with PVA incorporated in the internal matrix structure of the scaffold as PVA in the internal matrix has a better wetting property than scaffold without PVA (Sahoo et al., 2005). The SEM pictures (Figure 1a–d) have confirmed the four formulations of PLA scaffold showing a spherical shape with rough surface and covered with a thin film of polymer. The average diameter of different formulations of scaffold prepared in this study ranged from 145 μm to 162 μm (Table 1).
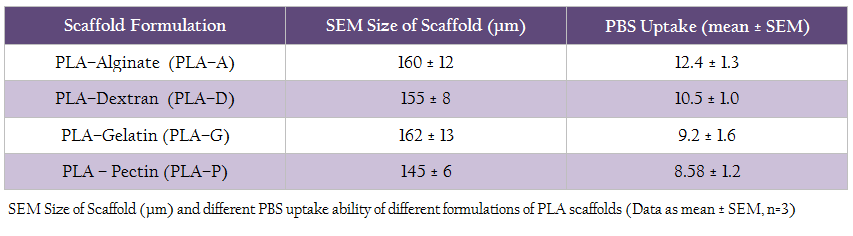
Table 1: SEM Size of Scaffold (µm) and different PBS uptake ability of different formulations of PLA scaffolds (Data as mean ± SEM, n=3)
The swelling ability of scaffold has vital significance during in vitro cell culture and for this, phosphate buffered saline (PBS, pH 7.2) was used for evaluating the PBS uptake ability of different scaffold formulations. All the scaffold formulations exhibited good swelling property and retained more PBS than their body weight. However, PLA–A scaffolds adsorbed more PBS compared to other formulations (Table 1).
Effect of Natural Polymer Blended PLA Scaffold Formulations on Growth of Fibroblast–Like Cells
The effect of natural polymer blended PLA scaffold on growth of fibroblast–like cells from heart of C. mrigala was studied 7–day post seeding. The seeded cells proliferated and spread with incubation time in all scaffold formulations, except the formulation containing pectin. Among the four formulated scaffolds, PLA–A and PLA–D scaffolds demonstrated significantly higher (p < 0.05) cell growth as compared to control and other formulations 7–day post seeding (figure 2). Based on the preliminary observations on scaffolds, their swelling properties and effect on the cell growth, PLA–A was further evaluated to determine its different seeding density effect on cell proliferation and growth.
Effect of PLA–A on the Growth and Proliferation of Fibroblast–Like Cells at Varying Cell Seeding Density
Effect of PLA–A on the growth and proliferation of fibroblast–like cells from heart of C. mrigala at various seeding density was investigated. Fibroblast–like cells at a seeding density of (0.4, 0.6, 0.8, 1.2 and 1.6 × 10 5 cells ml–1 media) were incubated with PLA– A scaffolds for 9 days and compared with 2–D culture (control). The kinetics of growth of these cells on 3rd, 5th, 7th and 9th day, at different initial cell seeding density, was evaluated and depicted in figure 3. With initial cell seeding density of 0.4 and 0.6 × 105 cells ml–1 media, cells proliferated slowly irrespective of scaffold composition even up to 9 days. On the contrary, with higher cell seeding density (0.8 to 1.6 × 10 5 cells ml–1 media), good proliferation was observed even on 3rd day of post seeding. When comparison was made between the PLA–A and control (2–D culture), at an initial cell seeding density of 2.0 × 10 5 cells ml–1 media, the proliferating cells on PLA–A scaffold on 5th day of post seeding showed almost same number of cells as observed in 2–D culture at 9th day of incubation (Figure. 3). The avidity of cells to adhere and proliferate was observed in PLA–A scaffold formulation and microscopic photograph of cell growth and proliferation on 3rd, 5th, 7th and 9th day in PLA–A was taken and depicted in figure 4. In comparative study, it was found that, even with a low initial seeding density (1.6 × 10 5 cells ml–1 media), cell yield was found to be 1.5 times more in 3–D culture using polymeric scaffold (PLA–A) as compared to two–dimensional (2–D) cell culture with seeding density (2.0 × 10 5 cells ml–1 media) at 9th day post seeding.
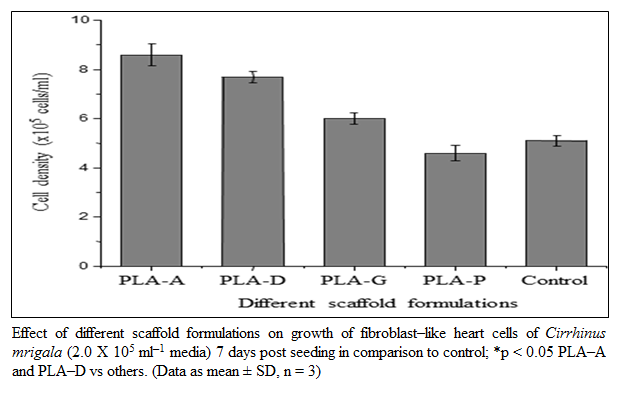
Figure 2: Effect of different scaffold formulations on growth of fibroblast–like heart cells of Cirrhinus mrigala (2.0 X 105 ml–1 media) 7 days post seeding in comparison to control; *p < 0.05 PLA–A and PLA–D vs others. (Data as mean ± SD, n = 3)
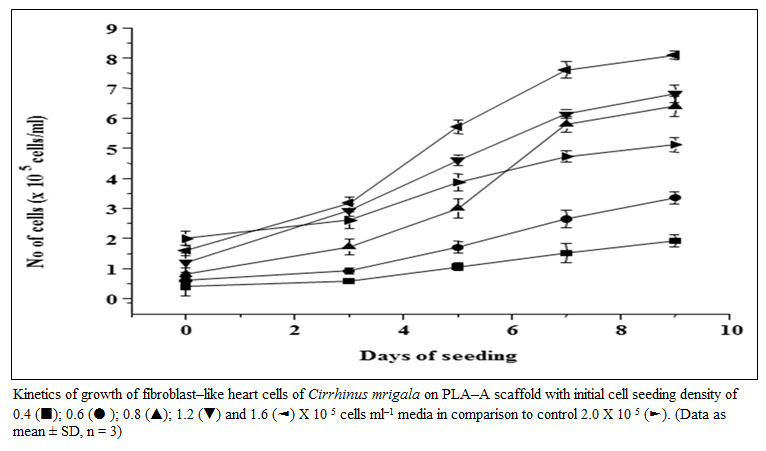
Figure 3: Kinetics of growth of fibroblast–like heart cells of Cirrhinus mrigala on PLA–A scaffold with initial cell seeding density of 0.4 (■); 0.6 (● ); 0.8 (▲); 1.2 (▼) and 1.6 (◄) X 10 5 cells ml–1 media in comparison to control 2.0 X 10 5 (►). (Data as mean ± SD, n = 3)
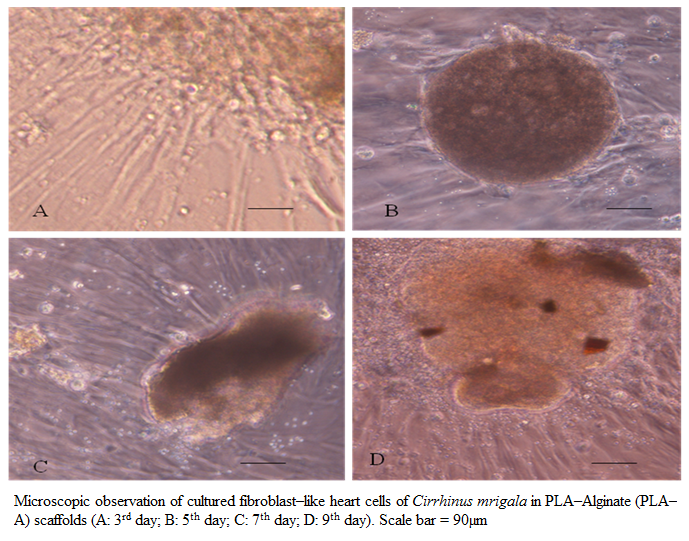
Figure 4: Microscopic observation of cultured fibroblast–like heart cells of Cirrhinus mrigala in PLA–Alginate (PLA–A) scaffolds (A: 3rd day; B: 5th day; C: 7th day; D: 9th day). Scale bar = 90µm
DISCUSSION
A paradigm shift is taking place to culture animal cells in 3–D within a polymeric matrix replacing the 2–D cell culture systems. Out of several polymers that are being used, PLA is the most commonly used because of its biocompatibility as well as high mechanical strength that maintains the matrix porous structure for cell migration and growth (Stock and Meyer, 2001, Cheng et al., 2009; Madhavan et al., 2010). Further, PLA polymers are used due to their relatively slower degradation rates as compared to other synthetic polymers (Wang et al., 2005). As PLA polymer is hydrophobic, coating or blending these polymeric scaffolds with hydrophilic polymers promotes cell adhesion (Lavik et al., 2002).
Further, till date no information is available on the use of scaffolds for evaluating their suitability in terms of adhesion, attachment, growth and proliferation of anchorage dependent fibroblast-like cells in fish cell culture. Keeping all these in mind, we fabricated porous PLA scaffolds blended with hydrophilic polymers of natural origin such as alginate (PLA-A), gelatin (PLA-G), pectin (PLA-P) and dextran (PLA-D) for this study. Further, PVA and chitosan were included in internal phase to promote adhesion. As PVA is amphiphilic in nature, the anchored PVA could have provided functional OH groups that have been reported as being helpful in cell attachment (Yang et al., 2002). In this study, chitosan was incorporated in the internal matrix structure for better cell growth of fibroblast–like heart cells of C. mrigala. Earlier, Chuang et al. (1999) have demonstrated that PVA membranes modified by blending with chitosan promote fibroblast adhesion.
The SEM studies have confirmed the four formulations of PLA scaffold showing a spherical shape with rough surface and covered with a thin film of polymer (figure 1). This rough surface could be due to adsorb property of chitosan onto the matrix interface because of its ionic interaction, as the scaffold polymer interface is anionic and chitosan is cationic (Sahoo et al., 2005). Moreover, the ability of chitosan to support cell attachment and survival has been attributed to its chemical structure, which resembles glycosaminoglycans, an extracellular matrix component (Mei et al., 2005). Further, sucrose, which is known to increase the surface tension of water (Lee and Timasheff, 1981), was used in the internal phase of the primary emulsion. This must have contributed in formation of larger water droplets and formed large void structures inside its matrix upon leaching out from scaffold materials. The porous matrix structure could have provided a greater surface area to promote cell adhesion, proliferation and growth as found in our study. Similarly, Particle size is an important parameter that could affect the degradation of the polymer matrix (Dunne et al., 2000). Therefore, to achieve scaffolds of desired diameter, the condition for emulsification and formulation composition was optimized.
The PBS uptake ability of polymeric scaffold mainly depends on the three–dimensional structure and hydrophilic nature of scaffold. It is very crucial to notice that the PBS (in replace of media) should adsorb properly into the scaffold matrix for influencing the biological activity of scaffold (Tan et al., 2007). Swelling of scaffold in culture condition increases the pore diameter, thus facilitating the cell not only to adhere but also to migrate in to the scaffolds; thus providing the scaffold the maximum internal surface area (Zhang and Zhang, 2001). The PBS uptake studies show that all the formulations exhibited good swelling capacity and retained more PBS than their body weight. However, PLA–A scaffold formulation showed more PBS retention as compared to other formulations. This variation in the extent of swelling and water retention ability could be due to the difference in hydrophilicity of the polymer chains and the cross–linking density (Xu and Burg, 2007).
PLA blended natural polymeric scaffolds were evaluated to find their suitability for in vitro growth of fibroblast–like cells. Fibroblast–like cells were chosen because of their high in vitro replication capacity and therefore, can be considered as an effective tool for preliminary studies of cell adhesion and growth onto a scaffold (Lazzeri et al., 2007). In our study, we observed that the cells seeded on PLA–A scaffold demonstrated better cell growth after 7th day post seeding as compared to other formulations as well as control. This could be due to the structural topography (many hydrophilic groups) and good medium retention ability of alginate. Because of this, the 3–D network of PLA–A could have retained large volume of nutrient medium and supported good swelling structure as observed by Peppas and Sahlin (1996) thus facilitating better proliferation of fibroblast–like cells.
Cell proliferation may differ depending on seeding density and scaffold type used. To ascertain this, PLA–A scaffold was taken to evaluate the effect of different seeding density on growth and proliferation of fibroblast–like cells since maximum proliferation was found in the above formulation. At lower initial cell seeding density (0.4 to 0 .6 × 10 5 cells ml–1 media), a lag phase in cell growth was recorded. This could be due to slow growth of cell for less cell–cell contact (Sahoo et al., 2005). Similarly, at high seeding density (1.6 ×10 5 cells ml–1 media), slow growth pattern was observed. This is in agreement with van Gaalen et al (2010) who also recorded that seeding more cells may not necessarily result in more proliferation. As with high seeding density, the scaffold surface might have been covered to its saturation capacity, and hence further cell growth may have been limited. The growth kinetics studies demonstrated that a seeding density of 0.8 to 1.6 × 10 5 cells ml–1 media showed good trend of proliferation compared to others. Hence, a substantial influence of the seeding density on growth and proliferation of fibroblast–like cell was observed on our formulated PLA–A scaffold as compared to 2–D culture (control).
This study revealed that among the four scaffold formulations, PLA–A could be potentially used for in vitro culture in achieving better growth and proliferation of anchorage dependent fibroblast– like cells from heart of Indian Major Carp, C. mrigala compared to 2–D cell culture. This opens up new possibilities for studying other complex biological processes in fish using 3–D culture.
ACKNOWLEDGEMENTS
The authors are thankful to the Director, Central Institute of Freshwater Aquaculture (ICAR), Kausalyaganga, Odisha, India and the Director, Institute of Life Sciences (DBT) for providing necessary facilities to carry out the present investigation.
REFERENCES
Abbott A (2003). Cell culture: Biology's new dimension. Nat. 424: 870–872.
http://dx.doi.org/10.1038/424870a
PMid:12931155
Carletti E, Motta A, Migliaresi C (2011). Scaffolds for tissue engineering and 3D cell culture. Methods Mol. Biol. 695: 17 – 39.
http://dx.doi.org/10.1007/978-1-60761-984-0_2
PMid:21042963
Cheng Y, Deng S, Chen P, Ruan R (2009). Poly lactic acid (PLA) synthesis and modifications: a review. Front. Chem. China 4: 259 – 264.
http://dx.doi.org/10.1007/s11458-009-0092-x
Chung S, King MW (2011). Design concepts and strategies for tissue engineering scaffolds. Biotechnol. Appl. Biochem. 58: 423 – 438.
http://dx.doi.org/10.1002/bab.60
PMid:22172105
Chuang WY, Young TH, Yao CH, Chiu WY (1999). Properties of the poly (vinyl alcohol)/chitosan blend and its effect on the culture of fibroblast in vitro. Biomaterials. 20: 1479 – 1487.
http://dx.doi.org/10.1016/S0142-9612(99)00054-X
Cui YL, Qi AD, Liu WG, Wang XH, Wang H, Ma DM, Yao KD (2003). Biomimetic surface modification of poly (l–lactic acid) with chitosan and its effects on articular chondrocytes in vitro. Biomaterials. 24: 3859 – 3868.
http://dx.doi.org/10.1016/S0142-9612(03)00209-6
Dhiman HK, Ray AR, Panda AK (2004). Characterization and evaluation of chitosan matrix for in vitro growth of MCF– 7 breast cancer cell lines. Biomaterials. 25: 5147 – 5154.
http://dx.doi.org/10.1016/j.biomaterials.2003.12.025
PMid:15109838
Dunne M, Corrigan I, Ramtoola Z (2000). Influence of particle size and dissolution conditions on the degradation properties of polylactide– co–glycolide particles. Biomaterials. 21(16): 1659 – 1668.
http://dx.doi.org/10.1016/S0142-9612(00)00040-5
Garrick RA (2000). Isolation and culture of capillary endothelial cells from the eel, Anguilla rostrata. Microvasc. Res. 59: 377 – 385.
http://dx.doi.org/10.1006/mvre.1999.2234
PMid:10792969
Harrington H, Rose FRAJ, Aylott JW, Ghaemmaghami AM (2013). Self–reporting scaffolds for 3–Dimensional cell culture. J. Vis. Exp. 7(81): e50608.
Lavik E, Teng YD, Snyder E and Langer R (2002). Seeding neural stem cells on scaffolds of PGA, PLA, and their copolymers. Methods Mol. Biol. 198: 89–97.
PMid:11951644
Lazzeri L, Cascone MG, Danti S, Serino LP, Moscato S, Bernardini S (2007). Gelatine/PLLA sponge–like scaffolds: morphological and biological characterization. J. Mater Sci. Mater Med. 18: 1399 – 1405.
http://dx.doi.org/10.1007/s10856-007-0127-0
PMid:17277980
Lee JC, Timasheff SN (1981). The stabilization of proteins by sucrose. J. Biol. Chem. 256: 7193 – 7201.
PMid:7251592
Madhavan NK, Nair NR, John RP (2010). An overview of the recent developments in polylactide (PLA) research. Bioresour. Technol. 101: 8493 – 8501.
http://dx.doi.org/10.1016/j.biortech.2010.05.092
PMid:20630747
Mei N, Chen G, Zhou P, Chen X, Shao ZZ, Pan LF, Chun GWV (2005). Biocompatibility of poly (epsilon–caprolactone) scaffold modified by chitosan–the fibroblasts proliferation in vitro. J. Biomater. Appl. 19: 323 – 339.
http://dx.doi.org/10.1177/0885328205048630
PMid:15788428
Mikos AG, Bao Y, Cima LG, Ingber DE, Vacanti JP, Langer R (1993). Preparation of poly (glycolic acid) bonded fiber structures for cell attachment and transplantation. J. Biomed. Mater. Res. 27: 183 – 189.
http://dx.doi.org/10.1002/jbm.820270207
PMid:8382203
Mikos AG, Thorsen AJ, Czerwonka LA, Bao Y, Langer R, Winslow DN, Vacanti JP (1994a). Preparation and characterization of poly (L–lactic acid) foams. Polymer. 35: 1068 – 1077.
http://dx.doi.org/10.1016/0032-3861(94)90953-9
Mikos AG, Lyman MD, Freed LE, Langer R (1994b). Wetting of poly (lactic acid) and poly (dl–lactic–co–glycolic acid) foams for tissue culture. Biomaterials. 15: 55 – 58.
http://dx.doi.org/10.1016/0142-9612(94)90197-X
Mooney DJ (1999). DNA delivery from polymer matrices for tissue engineering. Nat. Biotech. 17: 551 – 554.
http://dx.doi.org/10.1038/9853
PMid:10385318
Mooney DJ, Park S, Kaufmann PM, Sano K, McNamara K, Vacanti JP, Langer R (1995). Biodegradable sponges for hepatocyte transplantation. J. Biomed. Mater. Res. 29: 959 – 965.
http://dx.doi.org/10.1002/jbm.820290807
PMid:7593039
Mothersill C, Lyng F, Lyons M, Cottell D (1995). Growth and differentiation of epidermal cells from the rainbow trout established as explants and maintained in various media. J. Fish Biol. 46: 1011 – 1025.
http://dx.doi.org/10.1111/j.1095-8649.1995.tb01406.x
Murphy WL, Dennis RG, Kileny JL and Mooney DJ (2002). Salt fusion: an approach to improve pore interconnectivity within tissue engineering scaffolds. Tissue Eng. 8: 43–52.
http://dx.doi.org/10.1089/107632702753503045
PMid:11886653
Nanda PK, Swain P, Nayak SK, Dash S, Routray P, Swain SK, Patra BC (2009). Goat serum as an alternative to establish cell culture from Indian Major Carp, Cirrhinus mrigala. In Vitro Cell Dev. Biol. Anim. 45: 148 – 151.
http://dx.doi.org/10.1007/s11626-008-9160-7
PMid:19118441
Peppas NA, Sahlin JJ (1996). Hydrogels as mucoadhesive and bioadhesive materials: a review. Biomaterials. 17: 1553 – 1561.
http://dx.doi.org/10.1016/0142-9612(95)00307-X
Rasal RM, Janorkar AV, Hirt DE (2010). Poly (lactic acid) modifications. Prog. Poly. Sci. 35(3): 338 – 356.
http://dx.doi.org/10.1016/j.progpolymsci.2009.12.003
Sachlos E, Czernuszka JT (2003). Making tissue engineering scaffolds work review: the application of solid freeform fabrication technology to the production of tissue engineering scaffolds. Eur. Cell Mater. 5: 29 – 39.
PMid:14562270
Sahoo SK, Panda AK, Labhasetwar V (2005). Characterization of porous PLGA/PLA microparticles as a scaffold for three–dimensional growth of breast cancer cells. Biomacromolecules. 6: 1132 – 1139.
http://dx.doi.org/10.1021/bm0492632
PMid:15762686
Stock UA, Mayer JE (2001). Tissue engineering of cardiac valves on the basis of PGA/PLA co–polymers. J. Long Term Eff. Med. Implants 11 (3–4): 249 – 260.
PMid:11921667
Tan H, Gong Y, Lao L, Mao Z, Gao C (2007). Gelatin/chitosan/hyaluronan ternary complex scaffold containing basic fibroblast growth factor for cartilage tissue engineering. J. Mater. Sci. Mater. Med. 18: 1961 – 1968.
http://dx.doi.org/10.1007/s10856-007-3095-5
PMid:17554603
van Gaalen SM, de Bruijn JD, Wilson CE, van Blitterswijk CA, Verbout AJ, Alblas J, Dhert WJA (2010). Relating cell proliferation to in vivo bone formation in porous Ca/P scaffolds J. Biomed. Mater. Res. 92A: 303 –310.
http://dx.doi.org/10.1002/jbm.a.32380
PMid:19189390
Voytik–Harbin SL (2001). Three–dimensional extra cellular matrix substrates for cell culture. Methods Cell Biol. 63: 561 – 581.
http://dx.doi.org/10.1016/S0091-679X(01)63030-9
Wang S, Cui W, Bei J (2005). Bulk and surface modifications of polylactide. Anal. Bioanal. Chem. 381(3): 547 – 556.
http://dx.doi.org/10.1007/s00216-004-2771-2
PMid:15672238
Wolf K, Quimby MC (1976). Procedures for sub culturing fish cells and propagating fish cell lines. Tissue Cult. Assoc. Man. 24: 471 – 474.
http://dx.doi.org/10.1007/BF00918344
Xu F, Burg KJL (2007). Three–dimensional polymeric systems for cancer cell studies. Cytotechnol. 54: 135 – 143.
http://dx.doi.org/10.1007/s10616-007-9065-4
PMid:19003005 PMCid:PMC2267509
Yang J, Shi G, Bei J, Wang S, Cao Y, Shang Q, Yang G, Wang W (2002). Fabrication and surface modification of macroporous poly (l–lactic acid) and poly (l–lactic–co–glycolic acid) (70/30) cell scaffolds for human skin fibroblast cell culture. J. Biomed. Mater Res. 62: 438 – 446.
http://dx.doi.org/10.1002/jbm.10318
PMid:12209930
Zhang Y, Zhang M (2001). Synthesis and characterization of macroporous chitosan / calcium phosphate composite scaffolds for tissue engineering. J. Biomed. Mater. Res. 55: 304 – 312.
http://dx.doi.org/10.1002/1097-4636(20010605)55:3<304::AID-JBM1018>3.0.CO;2-J
Zhu H, Ji J, Lin R, Gao C, Feng L, Shen J (2002). Surface engineering of poly (D, L–Lactic acid) by entrapment of chitosan–based derivatives for the promotion of chondrogenesis. J. Biomed. Mater. Res. 62: 532 – 539.
http://dx.doi.org/10.1002/jbm.10313
PMid:12221701