Advances in Animal and Veterinary Sciences
Research Article
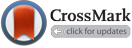
The Level of Endotoxin in Organs, Antibiotic Sensitivity, and Serotyping of Bacteria Isolated from Cats and Dogs with Septicaemia
Rathiymaler Maniam1, Faez Firdaus Jesse Abdullah2, Zunita Zakaria3, Mohd Zamri Saad1, Annas Salleh1*
1Department of Veterinary Laboratory Diagnosis, Faculty of Veterinary Medicine, Universiti Putra Malaysia, 43400 Serdang, Selangor, Malaysia; 2Department of Veterinary Clinical Studies, Faculty of Veterinary Medicine, Universiti Putra Malaysia, 43400 Serdang, Selangor, Malaysia; 3Department of Veterinary Pathology and Microbiology, Faculty of Veterinary Medicine, Universiti Putra Malaysia, 43400 Serdang, Selangor, Malaysia.
Abstract | Septicaemia is the presence of bacteria and its toxins in blood circulation, potentially leads to sepsis and death. Despite its importance in human medicine, study pertaining to septicaemia in veterinary medicine has been lacking. The study was conducted to measure the concentration of endotoxin in vital organs and their relationship with point of entry of septicaemia in dogs and cats with septicaemia. Subsequently, common septicaemic agents and their sensitivity towards antibiotics were determined. Fifty carcasses of cats and dogs were selected. Samples of the heart, lungs, liver, and kidney were collected for bacterial identification and endotoxin concentration quantitation. The three most commonly isolated bacteria were subjected to antimicrobial sensitivity testing using disc diffusion technique. As Escherichia coli was later determined as the most common bacteria, the isolates were subjected to in silico serotyping. It was observed that E. coli, Klebsiella pneumoniae, and Staphylococcus pseudintermedius were the most commonly isolated bacteria in cats and dogs with septicaemia. Endotoxin was detected from all of the collected organs, with significantly (p<0.05) high concentration of endotoxin in lungs and kidney when septicaemia originated from the respiratory tract or urinary tract, respectively. These findings were consistent in both cat and dog. Escherichia coli were sensitive to sulfamethoxazole/trimethoprim and enrofloxacin, and resistant to clindamycin (intrinsic resistance), K. pneumoniae were observed to be resistant towards sulfamethoxazole/trimethoprim, clindamycin (intrinsic resistance), cephalexin, enrofloxacin, and amoxicillin (intrinsic resistance). On the other hand, S. pseudintermedius were sensitive towards all of the tested antibiotics. In silico serotyping of E. coli revealed high percentage of serotype O104:H4 and O102:H18 which were associated with infections in human. E. coli is the most commonly isolated bacteria and the gastrointestinal is the most common point of entry for septicaemia in cats and dogs. Common septicaemic agents of these companion animals showed sensitivity and resistance towards antibiotics commonly used in veterinary practice.
Keywords | Septicaemia, Cat, Dog, Endotoxin, Antibiotic sensitivity
Received | March 09, 2020; Accepted | April 15, 2020; Published | May 15, 2020
*Correspondence | Annas Salleh, Department of Veterinary Laboratory Diagnosis, Faculty of Veterinary Medicine, Universiti Putra Malaysia, 43400 Serdang, Selangor, Malaysia; Email: annas@upm.edu.my
Citation | Maniam R, Abdullah FFJ, Zakaria Z, Saad MZ, Salleh A (2020). The level of endotoxin in organs, antibiotic sensitivity, and serotyping of bacteria isolated from cats and dogs with septicaemia. Adv. Anim. Vet. Sci. 8(6): 614-623.
DOI | http://dx.doi.org/10.17582/journal.aavs/2020/8.6.614.623
ISSN (Online) | 2307-8316; ISSN (Print) | 2309-3331
Copyright © 2020 Lukkananukool et al. This is an open access article distributed under the Creative Commons Attribution License, which permits unrestricted use, distribution, and reproduction in any medium, provided the original work is properly cited.
INTRODUCTION
Septicaemia is a condition where there is persistent presence of microorganism, usually bacteria, and its toxins in the blood circulation. This subsequently leads to overwhelming systemic inflammatory response by the host, termed as sepsis, which is potentially fatal. Septicaemia and sepsis have been extensively studied in the human medicine, but not in veterinary medicine. Septicaemia has been shown to be an important condition contributed to mortality of cats and dogs (Rathiymaler et al., 2017). Gram-positive bacteria is commonly associated with septicaemia in human (Ramachandran, 2014). On the contrary, Gram-negative bacteria are more common in animals with Escherichia coli being the most common pathogen in carcasses of septicaemic cats and dogs (Maniam et al., 2019; Zakaria et al., 2019)
Pathogenesis of septicaemia involves bacterial colonization in an organ prior to rapid release of endotoxin, followed by its entry into the blood circulation. This is followed by multiplication and further release of endotoxin to cause acute death of animals (Annas et al., 2015). Similar pathogenic mechanism could be employed by other septicaemic-causing Gram-negative pathogens in dogs and cats. Common points of entry of septicaemia are via the gastrointestinal, respiratory, integumentary and urinary systems (Maniam et al., 2019). Since different body systems are closely related with different vital organs, it is intriguing to investigate the role of endotoxin in various vital organs of septicaemic dogs and cats.
Treatment of septicaemia and sepsis principally focused towards eradication of the aetiological agent using antimicrobial agents, management of fever, respiratory stabilization, restoration of organ perfusion, and controlling inflammatory reactions (Gauer, 2013). In human medicine, despite the advancement in pharmacotherapy and supportive care, the mortality rates due to septic shock and sepsis remained high. While some antimicrobial drugs are effective in killing the pathogen, they may not be effective in removing endotoxin from the blood circulation. This has led to few studies in human and veterinary medicines aimed at assessing the removal or neutralization of endotoxin (Davies and Cohen, 2011). Other issue pertaining to antimicrobial usage in treatment of septicaemia is the development of antimicrobial resistance (AMR) (Lanz et al., 2003; Inglis and Urosevic, 2017).
This study was conducted to determine the common septicaemic agents of cats and dogs, concentration of endotoxin in vital organs and their relationship with point of entry, sensitivity of the common septicaemic agents towards commonly used antibiotics, and serotyping of the most common septicaemic agent.
MATERIALS AND METHODS
Study design
Carcasses of cats and dogs were obtained from the Post-mortem Laboratory, Faculty of Veterinary Medicine, Universiti Putra Malaysia, and from two veterinary clinics and one animal shelter in Klang Valley, Malaysia throughout the year 2017. Necropsy and sample collections were made only on carcasses that were examined in less than 18 hours after death. Samples of the heart, lungs, liver, and kidney were aseptically collected and were subjected to bacterial isolation and identification by routine biochemical tests. Cases from which similar bacteria were isolated in three or more samples were diagnosed as septicaemia. Subsequently, the point of entry of the microorganism was determined based on the severity of lesion and the post-mortem examination as previously described (Maniam et al., 2019). Those carcasses that were not diagnosed as septicaemia were excluded from the study. This screening process was done until a total of 50 septicaemia cases (25 cats and 25 dogs) were obtained. The collected organs were homogenized, and kept at -30°C while waiting for confirmation of septicaemia. These organs were later subjected to endotoxin quantitation. Treatment histories from cases diagnosed as septicaemia were traced to analysed the therapeutic history.
Bacterial isolation and identification
The organ samples were subjected to bacterial isolation as previously described (Aggrawal, 2014). Briefly, the surface of the organs was seared using a heated scalpel blade before incising the tissue. Sterile swabs were inserted into the tissues, and were cultured onto blood agar and incubated at 37°C for 24 hours. Growth of bacterial colonies with similar morphology from three or more organs was tentatively diagnosed as septicaemia. For confirmation of septicaemia, these bacterial colonies were subjected to routine biochemical identification as described (Carter and Cole, 2012). Briefly, the pure bacterial colonies were stained with Gram stain. The resultant Gram-negative bacteria were cultured on eosin methylene blue agar (EMBA) and MacConkey agar and incubated for 24 hours at 37°C, and subjected to biochemical tests of oxidase, triple sugar iron agar, sulphur indole motility, urease, and citrate tests. On the other hand, Gram-positive bacteria were subjected to catalase test. Catalase positive results were subjected to slide coagulation, hemolysin, VP, maltose, mannitol, and ADH tests, while catalase negative results were subjected to hemolysins, bile esculin, sucrose, glucose, maltose, and lactose tests. Results of the test were used to identify genus or species of bacteria according to previously described (Carter and Cole, 2012).
Quantitation of endotoxin in organs
Endotoxin concentrations in the lungs, liver, and kidney were determined using Limulus Amoebocyte Lysate (LAL) Assay (Pierce LAL Chromogenic Endotoxin Quantitation Kit, Thermo Scientific, USA) according to the manufacturer’s protocol as previously described (Annas et al., 2015). Briefly, endotoxin standard stock from E. coli provided in the kit was prepared to final endotoxin concentrations of 0.5 endotoxin unit (EU)/ml, 0.25 EU/ml, and 0.1 EU/ml. Microplates were heated for 10 minutes at 37°C before 50 µl of each endotoxin standard was dispensed into the microplate wells and incubated for 5 minutes at 37°C. Next, 50 µl of LAL was added into each well, gently shaken and incubated at 37°C for 10 minutes. This was followed by adding 100 µl of substrate solution into each well, gently shaken and incubated at 37°C for 6 minutes. Subsequently, 50 µl of stopping reagent (25% acetic acid) was added and gently shaken to stop the reaction. Absorbance was measured at 405 nm on a plate reader. The average absorbance of blank replicates was subtracted from the average absorbance of all individual endotoxin standards. Standard curves were prepared based on the endotoxin standards, and standard curve with coefficient of determination (r2) value of more than 0.98 were used.
Should a particular carcass was diagnosed with septicaemia, its homogenized organs were thawed and subjected to determination of endotoxin quantitation. Seventy-five µl of the homogenate were separated into sterile micro-centrifuge tube partially immersed in ice bath and 150 µl of 0.32 M perchloric acid was added to avoid presence of inhibitors to the lysate. The mixture was then incubated at 37°C for 20 minutes and centrifuged at 2000 rpm for 15 minutes. Then, the supernatant was added with equal volume of 0.18 M sodium hydroxide (NaOH). The resultant aliquot was subjected to endotoxin quantitation using similar protocol and standard curves as describe for the endotoxin standards. Using the standard curve, the value of the endotoxin was determined and expressed as EU/ml, and compared between different organs and different bacterial species.
Antimicrobial sensitivity test
Based on the bacterial identification, the three most commonly isolated bacteria in cases of septicaemia were selected. These bacteria were subjected to antimicrobial sensitivity test using the disc diffusion technique. Selection of antibiotics were made based on the commonly used antibiotics in small animal clinics and hospitals in Malaysia as well as based on recommended antibiotics by the Clinical and Laboratory Standards Institute CLSI VET (2018). A total of 6 antimicrobial agents of different concentrations were used; amoxicillin 10 µg, amoxicillin/clavulanic acid 30 µg, cephalexin 30 µg, enrofloxacin 5 µg, sulfamethoxazole/trimethoprim 25 µg and clindamycin 2 µg. The zone of inhibition was calculated in triplicate, and interpreted based on the CLSI VET (2018) guidelines into susceptible, intermediate, and resistant. Since the aim was to investigate sensitivity of common isolated bacteria against commonly used antibiotics, the intrinsic resistance of bacteria against certain antibiotics were not considered prior to testing.
In silico serotyping of E. coli
Since E. coli was eventually determined as the most common causative agent for septicaemia in cats and dogs, all isolates of E. coli were subjected to genomic serotyping as previously described (Jenkins et al., 2015; Joensen et al., 2015). Briefly, all bacterial isolates that have been identified as E. coli by biochemical test were prepared in three sets of pure colonies. They were subjected to complete chromosomal genome sequencing (Apical Scientific Sdn. Bhd. Malaysia). A FASTA database was constructed, and consisted of the assembled genome. This was then uploaded to Sero Type Finder gene database (Joensen et al., 2015), with E. coli as the selected organism, at 85% threshold for %ID, 60% of minimum length of nucleotide sequence. The database was based on the O-antigen processing system genes wzx, wzy, wzm, and wzt for in silico O typing and the flagellin genes fliC, flkA, flmA, flnA, and fllA for in silico H typing.
Statistical analysis
The significant differences between microorganism isolated and treatment intervention and antibiotic sensitivity testing were analysed using Pearson Chi-Square method. The endotoxin measurements of each organ from all the 50 cases were analysed using Kruskal-Wallis and Dunn’s multiple comparison test to find the relationship of endotoxin level compared to the point of entry and microorganism isolated.
RESULTS
History of antibiotic treatment
Among the 25 dogs with septicaemia, 17 (68%) were treated with antibiotics during hospitalization, significantly (p<0.05) higher than those 8 (32%) without antibiotic treatment 8 [Chi square test, X2 (1, N=25 = 88.3, p= 0.036)]. Among cats, 21 (84%) were treated with antibiotics, significantly (p<0.05) higher than the 4 (16%) without antibiotic intervention [Chi square test, X2 (1, N=25= 99.2, p= 0.018)].
Bacterial isolation and identification
For cats that were diagnosed with septicaemia, 3 (12%) cases yielded pure isolation of E. coli whereas the other 22 (88%) cases had a mixture of 2 or more types of bacteria. Escherichia coli was commonly isolated from cats at 68%, followed by K. pneumoniae and S. pseudintermedius at 48% and 36%, respectively. However, no significant (p>0.05) different was observed between the type of bacterial isolation in cats.
As for the dogs, all 25 cases had mixed infection of 2 or more bacteria. Isolation of E. coli was significantly (p<0.05) more frequent, involving 84% of the cases compared to other bacteria. Similar to cats, this was followed by Klebsiella pneumoniae and Staphylococcus pseudintermedius at 56% and 44%, respectively. Summary of bacterial isolation from the cats and dogs are summarised in Table 1.
Table 1: Total number of each microorganism isolated with their respective percentages among the septicaemic cats and dogs.
Microorganism | Number of cases in cats / % | Number of cases in dogs / % | Total |
Escherichia coli | 17 (68%) | 21 (84%)* | 38 |
Klebsiella pneumoniae | 12 (48%) | 14 (56%) | 26 |
Staphylococcus pseudintermedius | 9 (36%) | 11 (44%) | 20 |
Staphylococcus intermedius | 8 (32%) | 8 (32%) | 16 |
Acinetobacter baumannii | 7 (28%) | 7 (28%) | 14 |
Streptococcus canis biotype 3 |
1 (4%) | 10 (40%) | 11 |
Enterococcus faecium | 2 (8%) | 8 (32%) | 10 |
Enterobacter cloacae | 4 (16%) | 5 (20%) | 9 |
Pseudomonas aeruginosa | 0 | 7 (28%) | 7 |
Enterococcus faecalis | 2 (8%) | 4 (16%) | 6 |
Pasteurella multocida | 3(12%) | 3 (12%) | 6 |
Streptococcus canis biotype 2 |
5 (20%) | 0 | 5 |
Aeromonas sp. |
0 | 5 (20%) | 5 |
Rhodococcus equi | 5 (20%) | 0 | 5 |
Streptococcus sp. |
1 (4%) | 4 (15%) | 5 |
Pasteurella sp. |
2 (8%) | 2 (8%) | 4 |
Streptococcus viridians | 1 (4%) | 2 (8%) | 3 |
Streptococcus sp. |
1 (4%) | 0 | 1 |
Proteus mirabilis | 0 | 1 (4%) | 1 |
Total | 80 | 112 | 192 |
Chi-square test, X2 (15, N=25) = 23.8, p=0.038. *E. coli is significantly isolated compared to other bacteria among septicaemic dogs. Chi-square test, X2 (15, N=25) = 52.9, p=0.471, there is no significant difference of between these types of bacteria causing septicaemia among the cats.
Table 2: Mean ±SD concentrations of endotoxin in organs and points of entry in cats and dogs diagnosed with septicaemia.
Animal | Points of Entry | Concentration of endotoxin in organs (EU/ml) | |||
Liver | Lung | Kidney | Heart | ||
Cat | Respiratory | 3.16± 1.36 | 4.67± 1.50* | 0.92± 0.56 | 1.58± 0.93 |
Gastrointestinal | 3.69± 0.81 | 2.25± 0.72 | 1.19±=0.63 | 1.21± 0.21 | |
Integumentary | 3.28±1.82 | 1.92± 0.62 | 1.12±0.71 | 1.54± 0.47 | |
Urinary | 1.43±1.03 | 1.60± 1.07 | 3.92± 0.84* | 1.55± 0.83 | |
Total | 11.5483 | 10.4375 | 7.16 | 5.88 | |
Dog | Gastrointestinal | 4.29± 1.2* | 1.47± 0.74 | 1.33± 0.51 | 1.50± 0.75 |
Integumentary | 2.80± 0.91 | 1.20± 1.71 | 0.90± 0.83 | 1.21± 0.39 | |
Urinary | 2.58± 0.67 | 1.45± 0.76 | 4.09± 0.88* | 1.74± 0.71 | |
Respiratory | 2.18± 0.34 | 4.61±1.24 | 1.30± 0.62 | 1.47± 0.27 | |
Reproductive | 2.73± 0.27 | 0.74± 0.76 | 3.59±1.43 | 1.89± 0.56 | |
Musculoskeletal | 4.29± 0.69 | 1.07± 0.34 | 1.00± 0.11 | 2.3± 0.96 | |
Total | 18.87 | 10.543 | 12.22 | 10.19 |
* indicates significance difference at p<0.05.
ndotoxin concentrations in organs
In general, endotoxin was detected in all organs of all cases of septicaemia in dogs and cats. Significant (p<0.05) difference in the endotoxin concentration was observed between the four organs of both cats [F (3,21) = 9.01, p=0.029] and dogs [F(3, 21) = 7.53, p= 0.031]. In cats, the endotoxin concentration in the liver (3.0EU/ml±0.31) was significantly (p<0.05) higher than the kidney (1.52EU/ml±0.36) and heart (1.45EU/ml±0.13). The endotoxin concentration in the lung (2.96EU/ml±0.29) was not significantly (p>0.05) different to the other three organs. In dogs, the concentration of endotoxin in liver (3.70EU/ml±0.55) was significantly (p<0.05) higher than the lung (1.51EU/ml±0.72), kidney (1.67EU/ml±0.81) and heart (1.53EU/ml±0.35).
Association between endotoxin concentration in organs and point of entry of septicaemia
Significant (p<0.05) differences were observed between the respiratory, gastrointestinal, integumentary, and urinary systems for cats. In dogs, only gastrointestinal, integumentary, and urinary systems were observed, as musculoskeletal and reproductive systems were involved in only one case (Table 2).
In cats, statistical analysis showed significant (p<0.05) difference in the endotoxin concentration in the lungs when septicaemia was originated from the respiratory system compared to gastrointestinal, integumentary, and urinary systems. Significant (p<0.05) difference was also observed in the endotoxin concentration of the kidney in cases where septicaemia was originated from the urinary system, compared to other points of entry. No significant (p>0.05) difference was observed for the endotoxin concentration in the liver and heart with any of the points of entry (Table 2).
In dogs, significant (p<0.05) difference was observed in the endotoxin concentration of the liver in cases where septicaemia was originated from the gastrointestinal system compared to the urinary, or integumentary systems. The concentration of endotoxin in the kidney was significantly (p<0.05) higher in cases when septicaemia originated from the urinary system compared to other points of entry. However, no significant (p>0.05) difference was noted for the concentration of endotoxin in the lungs and heart with any point of entry (Table 2).
Association between endotoxin concentration in organs and isolated bacteria
No significant (p>0.05) difference was observed between the bacteria isolated and endotoxin concentrations in both dogs [F (1, 23) = 11.63, p= 0.791] and cats [F (2, 22) = 13.71, p= 0.827]. Thus, no significant association among the endotoxin concentration could be concluded according to the types of bacteria isolated. However, in both dogs and cats, total endotoxin concentrations were highest in cases with two or more Gram-negative bacterial isolations compared to pure isolation of E. coli and cases with mixture of Gram-negative and Gram-positive bacterial isolations (Table 3).
Antibiotic sensitivity test
The antibiotic sensitivity testing revealed that 100% and 63.2% of the E. coli isolated from cats and dogs were sensitive to sulfamethoxazole/trimethoprim and enrofloxacin, respectively. This was significantly (p<0.05) higher compared to other tested antibiotics. Furthermore, 94% of the E. coli isolates were resistant to clindamycin, which was significantly (p<0.05) higher compared to the other tested antibiotics. The E. coli isolated from dogs was significantly (p<0.05) more sensitive towards amoxicillin compared to those isolated from cats (Table 4).
As for the K. pneumoniae isolates, high percentage of resistance was observed towards sulfamethoxazole/trimethoprim (88.5%), clindamycin (88%), cephalexin (84.6%), enrofloxacin (84.6%), and amoxicillin (76.9%), which was significant (p<0.05) compared to amoxicillin/clavulanic acid (Table 4).
For Staphylococcus pseudintermedius, the isolates were significantly (p<0.05) sensitive to all of the antibiotics tested, with insignificant (p>0.05) difference for intermediate sensitivity and resistance to all of the tested antibiotics (Table 4).
In silico serotyping of E. coli
Genomic sequencing of E. coli from the carcasses of septicaemic cats revealed that the most common serotype of E. coli isolated was O2:H6, followed by O179:H9. On the other hand, in dogs, E. coli of serotype O104:H4 and O102:H18 were most commonly isolated, followed by the serotype O89:H9 and O6:H31 (Table 5).
Discussion
This study highlights the common bacterial species that cause septicaemia in cats and dogs, where E. coli, K. pneumoniae, and Staphylococcus pseudintermedius were identified as the three most commonly isolated bacteria. As previously observed, Gram-negative bacteria represent the major group of pathogen causing septicaemia in cat and dog. This group of bacteria possess endotoxin (Ramachandran, 2014) known to be an integral contributing factor for causing lethal shock in human and animals (Osterbur et al., 2014). It is most likely that a large portion of bacterial septicaemia is attributed to Gram-negative bacteria due to their capability to survive and to impair the host defence. Both of which are brought about by their endotoxin (Jan, 2017; Khan et al., 2018). On the other hand, Gram-positive bacteria lacks endotoxin, thus the endotoxin concentrations were consistently low in cases of septicaemia by mixed infection by Gram-negative and Gram-positive bacteria.
Table 3: Mean±SD concentrations of endotoxin in organs and the microorganism isolated from cats and dogs with septicaemia
Animal | Bacteria isolation | Types of bacteria | Concentration of endotoxin in organs (EU/ml) | Total | |||
Liver | Lung | Kidney | Heart | ||||
Cat | Single | Escherichia coli | 1.62± 0.96 | 1.29± 1.21 | 3.07± 1.18 | 1.13± 0.14 | 7.11 |
Mixture | Gram-negative only | 3.49± 0.32 | 3.47± 0.64 | 1.44± 0.79 | 1.54± 0.45 | 9.93 | |
Gram-negative and Gram-positive | 2.95± 0.44 | 2.79± 0.71 | 1.13± 0.54 | 1.43± 0.87 | 8.31 | ||
Dog | Mixture | Gram-negative only | 4.32± 1.54 | 1.46± 0.76 | 1.70± 0.66 | 1.65± 0.86 | 9.12 |
Gram-negative and Gram-positive | 2.91± 0.75 | 1.57± 0.99 | 1.64± 0.34 | 1.38± 1.72 | 7.50 |
Table 4: Antibiotic sensitivity of bacteria isolated from carcasses of cats and dogs with septicaemia to commonly used antibiotics.
Bacteria
|
Sensitivity | Percentage of isolates and their antibiotic sensitivity (%) [number of samples for cat, dog] | |||||
Amo | Amo/Cla | Cep | Enro | Sul/Tri | Cli | ||
E. coli (n=38)
|
Sensitive | 52.6 | 44.7 | 36.8 | 63.2* | 100* | 6.0 |
[4,16*] | [7,10] | [5,9] | [10,14] | [17,21] | [0,2] | ||
Intermediate | 0 | 2.6 | 13.2 | 31.6 | 0 | 0 | |
[0,0] | [0,1] | [1,4] | [5,7] | [0,0] | [0,0] | ||
Resistant | 47.4 | 52.6 | 50.0 | 5.2 | 0 | 94.0 | |
[13,5*] | [10,10] | [11,8] | [2,0] | [0,0] | [17,19] | ||
K. pneumoniae (=26)
|
Sensitive | 19.2 | 0 | 0 | 0 | 3.9 | 0 |
[2,3] | [0,0] | [0,0] | [0,0] | [0,1] | [0,0] | ||
Intermediate | 3.9 | 50.0 | 15.4 | 15.4 | 7.6 | 2.0 | |
[0,1] | [7,6] | [0,4] | [2,2] | [0,2] | [1,2] | ||
Resistant | 76.9* | 50 | 84.6* | 84.6* | 88.5* | 88* | |
[10,10] | [5,8] | [12,10] | [10,12] | [12,11] | [11,12] | ||
S. pseud-intermedius (n=20) |
Sensitive | 100* | 100* | 100* | 80.0* | 80.0* | 75.0* |
[9,11] | [9,11] | [9,11] | [7,9] | [9,7] | [6,9] | ||
Intermediate | 0 | 0 | 0 | 20.0 | 15.0 | 10.0 | |
[0,0] | [0,0] | [0,0] | [2,2] | [0,3] | [1,1] | ||
Resistant | 0 | 0 | 0 | 0 | 5.0 | 15.0 | |
[0,0] | [0,0] | [0,0] | [0,0] | [0,1] | [2,1] |
* indicates significance difference at p<0.05 between different antibiotics, or between cat and dog. Amo: amoxicillin; Amo/Cla: amoxicillin/clavulanic acid; Cep: cephalexin; Enro: enrofloxacin; Sul/Tri: sulfamethoxazole/trimethoprim; Cli: clindamycin. Green shaded areas indicate suggested groupings of antimicrobial agents; yellow shaded areas indicate groupings of antimicrobial agents not suggested by CLSI VET.
Table 5: In silico genomic serotyping of E. coli isolated from septicaemic carcasses of cats and dogs
Species of origin | Strain | Cases (%) | Serotype |
Cat |
E. coli strain Mt1B1 |
5(29.4%) | O2:H6 |
E. coli strain AR434 |
4(23.5%) | O179:H9 | |
E. coli strain AR436 |
2(11.8%) | O13:H4 | |
E. coli FDAARGOS 144 chromosome |
2(11.8%) | O13/O135:H4 | |
E. coli STEC299 |
2(11.8%) | O102:H18 | |
E. coli 2015C-4136CT1 |
2(11.8%) | O145:H34 | |
Dog | E. coli O104:H4 strain LB226692 | 5(23.8%) | O104:H4 |
E. coli STEC299 | 5(23.8%) | O102:H18 | |
E. coli strain AR435 | 3(14.3%) | O89:H9 | |
E. coli strain K-15KW01 | 3(14.3%) | O6:H31 | |
E. coli RM4715 | 2(9.5%) | O145:H34 | |
E. coli strain AR437 | 1(4.8%) | O8:H21 | |
E. coli BH100N substrain MG2017 | 1(4.8%) | ONT:H31 | |
E. coli ECCRA-119 chromosome | 1(4.8%) | O103:H25 |
In this study, most dogs and cats that succumbed to bacterial septicaemia were previously treated with antibiotics. Treatment using antibiotics has been postulated to results in sudden release of endotoxin into the host’s circulation due to the killing of the bacteria (Holzheimer, 2001). This further aggravates toxaemia and sepsis. Furthermore, the endotoxin may affect the host acutely prior to administration of treatment. It was found that the endotoxin acts before bacterial proliferation and causes acute kidney injury (Bellomo et al., 2017; Annas et al., 2015). The endotoxin is capable of causing apoptosis to the endothelial cells (Ramachandran, 2014), leading to sudden death associated with multiple organs dysfunction (Simmons and Pittet, 2015). In this study, however, the types of antibiotic used were not entirely traceable for analysis.
Analysis of the relationships between endotoxin concentrations in vital organs and points of entry for microorganism showed that endotoxin concentrations are highest in an organ adjacent to the point of entry. For example, concentrations of endotoxin in the liver of dogs are highest when septicaemia was originated from the gastrointestinal system. Similar findings were observed in the kidney and urinary tract, and the lungs and respiratory tract. The liver receives and filters blood from the gastrointestinal system. Thus when septicaemia originated from this system, the endotoxin is absorbed into the blood circulation and accumulates in the liver. The liver plays an important role in activation of Kupffer cells and neutralization of bacterial endotoxin (Gaddam et al., 2017), but this also directly increases the build-up of endotoxin in liver that leads to liver damage (Bode and Bode, 2005; Nolan, 2010). In cats, the endotoxin concentration in the liver is the highest when septicaemia was originated from the gastrointestinal system, but statistically insignificant. It is intriguing whether the liver of cats is more efficient in endotoxin neutralization compared to those of dogs.
The respiratory, gastrointestinal, and urinary systems are made up of tracts with orifices exposed to the external environment. Parts of these tracts harbour opportunistic pathogen (Nmema, 2017; Klepikov, 2019). The effect of endotoxin or septicaemia from one of these tract could lead to failure of the vital organs associated with it should no treatment is provided. Stress and immunosuppression, lower urinary tract diseases of cats, normal flora of the respiratory system such as Pasteurella multocida, and gastrointestinal diseases are some major risk factors that could lead to infections in these body systems (Lekcharoensuk et al., 2001; Puspitasari et al., 2018). Infection in these tracts would could lead to septicaemia leading to death as observed in this study.
In this study, it was discovered that E. coli was only significantly sensitive to sulfamethoxazole/trimethoprim and enrofloxacin. Antimicrobial resistance (AMR) has been a major issue as many bacterial pathogens are multidrug resistant (MDR) microorganism. This occurred largely due to inappropriate usage of antimicrobial drugs and the capability of each bacterial pathogen to escape the antimicrobial mechanism (Zowawi, 2016). In most veterinary practices, antimicrobial sensitivity test is not being practiced, which could contribute to AMR. If a bacterial pathogen is resistant to the used antimicrobial agent, outcome of the patient would not be improved even by early initiation of antibiotic treatment (Minasyan, 2017). Most commonly studied bacterial pathogens are Salmonella sp and methicillin resistant Staphylococcus aureus (MRSA) due to their public health concern (Murphy et al., 2009). However, throughout the years, E. coli has become a major concern too as it is a common enteric microflora that may be opportunistic pathogen (McInnes et al., 2020). It can potentially spread the resistant genes from human to animal and vice versa (Murphy et al., 2009). Out of the six antibiotics commonly used in small animal practices, E. coli are sensitive to only two antibiotics. Despite the sensitivity towards enrofloxacin, previous study reported that E. coli isolated from dogs with urinary tract infection was resistant to enrofloxacin (McMeekin et al., 2017). Improper usage of antibiotics have led the uprising of extended spectrum β-lactamase producing E. coli in countries including India (Chaudhuri et al., 2011), South Korea (Park et al., 2014), Thailand (Kanoksil et al., 2013), Cambodia (Vlieghe et al., 2015), Turkey (Saltoglu et al., 2015) and Romania (Hristea et al., 2015) that are resistance to aminoglycosides, quinolone, tetracyclines and sulfamethoxazole, and trimethoprim (Livermore, 2012; Akova, 2016).
Klebsiella pneumoniae isolated in this study were not sensitive to all of the antibiotics tested. Out of the six antibiotics, K. pneumonia was significantly resistant to five antibiotics. Similar observation was previously made by the World Health Organization, US Centres for Disease Control and Prevention, and the UK Department of Health (Kidd et al., 2017). Klebsiella pneumoniae was identified as a potential zoonosis threat (WHO, 2014) as it is an important cause of MDR infection worldwide (Kidd et al., 2017). However, the opposite result was seen for S. pseudintermedius. It was significantly sensitive to all six antibiotics tested. Hitherto, there were cases reported in human with infection of methicillin-resistant S. pseudintermedius (MRSP) ST17 with contact of dog, cat, and horses (Feßler et al., 2018). It was reported that currently the MRSA and MRSP have similar number of incidence and this is an alarming issue especially among the dog’s population (Murphy et al., 2009). Even though in our study the S. pseudintermedius was sensitive towards all of the antibiotics, precautions should be placed when using antibiotics to prevent MDR among S. pseudintermedius.
To date, limited studies have been conducted to highlight the importance of certain strains or serotypes of E. coli in causing diseases in animals including for septicaemia and sepsis. Previous study concluded that extraintestinal pathogenic E. coli (ExPEC) were more important than other strains in causing infection in cats and dogs with few of these isolates showing antimicrobial resistance (Osugui et al., 2014). In this study, the most commonly isolated strain of E. coli from dogs were E. coli O104:H4 strain LB226692 and E. coli STEC299 O102:H18. The latter strain was also isolated in 11.8% of the septicaemia cases of cats. In the year 2011, E. coli O104:H4 was found to be the causative agent of a disease outbreak involving more than 3000 persons in Germany (Loman et al., 2012). This strain is known to be of an enteroaggregative E. coli lineage. Interestingly, similar E. coli strain was isolated in 20% of the septicaemia case of dogs. On the other hand, E. coli STEC299 is a Shiga-toxin-producing E. coli and the same strain was recently found to be able to produce a novel Shiga toxin 2 subtype (Bai et al., 2018). STEC is long-known to cause severe gastrointestinal infection in human. On the other hand, E. coli Mt1B1 identified in 24% of the cases of cats was previously linked as intestinal isolate of mouse origin. The genomic identification of E. coli from cases of septicaemia from dogs and cats highlights the public health concerns of some of these isolates.
Conclusions
Escherichia coli was commonly isolated from carcasses of cats and dogs with septicaemia. Gram-negative bacteria were isolated in all cases, with gastrointestinal tract as the common point of entry leading to the liver having the highest concentration of endotoxin. Septicaemic agents of dogs and cats have certain degree of sensitivity and resistance towards commonly used antibiotics in small animal clinics. Some commonly isolated bacteria from septicaemic cases shows intrinsic resistance towards the commonly used antibiotics.
Acknowledgements
The authors would like to acknowledge Universiti Putra Malaysia for funding the study. Putra Grant: Inisiatif Pensyarah Muda (IPM) (9500900).
Authors Contribution
RM conducted the study, data analysis, and preparation of manuscript. FFJA, ZZ, and MZS revised the manuscript. AS designed and supervised the study, and revised the manuscript.
Conflict of interest
The authors declare that there is no conflict of interest regarding the publication of this article
References