Advances in Animal and Veterinary Sciences
Research Article
Evaluations of Potential Antiviral Effects of Green Zinc Oxide and Silver Nanoparticles against Bovine Herpesvirus-1
Gamil SG Zeedan1*, Khaled A Abd EL-Razik2, Ahmed M Allam1, Abeer M Abdalhamed1, Hala A Abou Zeina1
1Parasitology and Animals Diseases Department, National Research Centre,33 Bohouth St., Dokki, Giza, P.O. box 12622, Egypt; 2Reproduction diseases department, National Research Centre, 33 Bohouth St., Dokki, Giza, P.O. box 12622, Egypt.
Abstract | Viral infection is one of the most important life-threatening animals health. The emergence of new viral disease increases the demand for new therapeutic drugs. Current antiviral drugs have certain limitations for treating viral infection. The objective of the present study was aimed to use the plant extracts of olive leaves and natural honey for green synthesis of zinc oxide (ZnONPs) and silver nanoparticles (AgNPs) and investigate potential antiviral activities against bovine herpesvirus-1(BoHV-1) in vitro and in vivo. The formation of green NPs was confirmed by visible color change, transmission electron microscope (TEM), and UV- spectrophotometer. For determining ZnONPs and AgNPs cytotoxicity on MDBK cell culture using modified enzyme-linked immunosorbent assays (ELISA) and cytopathic effects (CPEs) to monitor the cytopathogenic changed. The antiviral activity of the green nanoparticles was evaluated using MDBK cell culture and experimental animals. The early signs observed that ZnONPs and AgNPs formation were color change from whitish to brownish color. The Transmission electron microscopy (TEM) analysis of NPs showed spherical nanoparticles and prismatic shapes showed size ranges between 10 nm to 50 nm. The UV- spectrophotometer analysis showed an absorption peak at 415 to 450 nm. Green ZnONPs and AgNPs are safe in MDBK cell culture at concentration ranged from 24 μg/mL to 50 μg /mL and they were protected the MDBK cell culture from BoHV-1 infection at nontoxic concentrations 25 μg/mL Green ZnO and AgNPs were safe in MDBK cell culture and in experimental animals. Although, the highest concentrations of ZnONPs and AgNPs cytotoxic effect with different degrees. ZnO and AgNPs protected the MDBK cell culture and experimental rabbits from experimentally infected with BoHV-1 infection when treating cell culture with green ZnONPs and AgNPs suspension. However, molecular redetection by polymerase chain reaction (PCR) was able to detect BoHV-1 in nasal swabs collected from control positive rabbit groups at the first 2 days after infection but failed to detect virus in treated groups with green ZnONPs and AgNPs suspension. Green ZnO and AgNPs can use in vitro and in vivo to protect cell culture and animals against BoHV-1viral infections. ZnO and AgNPs nontoxic and they are able to inhibit BoHV-1.
Keywords | BoHV-1, Honey, Olive leave plant extract, Silver Nanoparticles, Zinc oxide nanoparticles
Received | January 05, 2020; Accepted | March 24, 2020; Published | March 25, 2020
*Correspondence | Gamil Sayed Gamil Zeedan, Parasitology and Animals Diseases Department, National Research Centre,33 Bohouth St., Dokki, Giza, P.O. box 12622, Egypt; Email: gamilzee@yahoo.com
Citation | Zeedan, GSG, EL-Razik KAA, Allam AM, Abdalhamed AM, Zeina HAA (2020). Evaluations of potential antiviral effects of green zinc oxide and silver nanoparticles against bovine herpesvirus-1. Adv. Anim. Vet. Sci. 8(4): 433-443.
DOI | http://dx.doi.org/10.17582/journal.aavs/2020/8.4.433.443
ISSN (Online) | 2307-8316; ISSN (Print) | 2309-3331
Copyright © 2020 Zeedan et al. This is an open access article distributed under the Creative Commons Attribution License, which permits unrestricted use, distribution, and reproduction in any medium, provided the original work is properly cited.
INTRODUCTION
Bovine alpha herpesvirus-1 (BoHV-1) belongs to Varicellovirus genus under the family Herpesviridae, BoHV-1 is a highly contagious viral disease which causing infectious bovine rhinotracheitis in cattle (Biswas et al., 2013; Ranganatha et al., 2013). The BoHV-1 is responsible for significant economic losses in cattle and buffaloes industry (Biswas et al., 2013; Chandranaik et al., 2014; Kathiriya et al., 2018). There are no antiviral agents available capable of complete elimination of viral infection and facilitating recovery from latent infection. Although, similarity between BoHV-1 and human herpesvirus-1 (HHV-1) in pathogenicity, but they have a short replication cycle and ability to cause life-long infection (Nandi et al., 2009). BoHV-1establishes latency in nerve ganglia or tonsils, following primary nasal infection, or in the sacra ganglia, following genital infection and can periodically reactivated (Petrini et al., 2019). BoHV-1 is used for disease model to improve control strategies against herpesviruses infection in human and animals (Rasheed et al., 2018). BoHV-1vaccines are effective for reducing clinical signs and provided suboptimal protection against BoHV-1 in cattle (Domingo et al., 2019). Although, remarkable improved of antiviral agents by time to time but still need to search for board antiviral agents. Therefore, necessary developing antiviral agents targeting on infected cells for clarification from infectious virus in host organs, particularly in reservoir which spreading virus throughout animal herds (Xu et al., 2018). The available synthetic drugs possess several disadvantaged including toxicity and developed drug resistance (Chou and Lurain, 2019).
Recently, Nanotechnology is one of the most active research area associated with antiviral agents (Gao et al., 2018). In recent years, nanoparticles (NPs) and their oxides have unique properties could attribute to small sizes and large surface areas such as zinc and silver nanoparticles (Jeevanandam et al., 2018). There are many conventional methods for synthesis of zinc oxide (ZnONPs) which require high temperature-pressure conditions, and non-ecological chemicals (Ong et al., 2013). In recent years, the green synthesis has been gain attention, which eliminates the use of toxic chemical materials and applies ecofriendly which provided strategies of NPs green synthesis using plant extracts, biomolecules, and ionic liquids with low-temperature processes. A promising antiviral agents development lies in exploiting virus entry into cells through various cellular membrane receptors such as antiviral materials are silver and zinc oxide nanoparticles (Shamaila et al., 2016). The uniquely designed zinc oxide tetrapod structures contain engineered oxygen and therefore, highly negatively charged. They block infection of cultured cells by BoHV-1 (Shamaila et al., 2016).
Silver nanoparticles used in many fields as food technology, agriculture, medicine, and environmental technology (Vijayakumar et al., 2013; Dos Santos et al., 2014). In medical field, AgNPs considered novel therapeutic agents that widely recorded as antimicrobial agents, (Franci et al., 2015). Also, attracting attentions for their potential antiviral action, and is much research effort in the treatment of viral diseases (El-Mohamady et al., 2018). Against monkey poxvirus (El-Sayed and Kamel, 2018). Some synthesized antiviral compounds with other health hazards. In Egypt, the utilization of nanotechnology in human and veterinary medicine has shown a great growth. Nanotechnology holds a big promise for animal health, veterinary medicine and many areas of animal production (Nagajyothi et al., 2019). In this study, in vitro and vivo determination of the inhibitory effect of ZnO and AgNPs on Bovine herpesvirus-1 experimentally infected rabbits.
MATERIALS AND METHODS
Ethical statement
The study was conducted according to Medical Research Ethical Committee Research, National Statement on Ethical Conduct in Human and animals Research at National Research Centre, Egypt under registration # 19-135#.
Nanoparticles preparation
Synthesis of green zinc oxide and silver nanoparticles
It based synthesis of green ZnONPs on the biosynthesis method (Nagajyothi et al., 2019), with some modifications. Briefly; olive plant green leaves were washed with sterile distilled water, air dried, cut into small pieces and mixed with 250 ml of boiled deionized water. Zinc nitrate (Zn(NO3)2 · xH2O) purchased from Sigma-Aldrich (USA) dissolved in distilled water under constant stirring using magnetic stirrer and added after complete dissolution to Olive plant leaves extract. They kept the mixtures under stirring at 90°C for 5h. The results mixture centrifuged at 8000 rpm for 25 min, the sediment pale brown granules ZnONPs centrifuged filtered and dried at 80 °C for 7-8 h. Silver nanoparticles synthesis using natural local Egyptian honey: Fresh natural honey purchased from market at Cairo, Egypt. Silver nitrate (AgNO3) purchased from Sigma-Aldrich (USA). Honey used different pH according to the method described (Winn, 2006). Honey weights were 30 and 40 g dissolved in 80 mL, 70 and 60 mL de-ionized water, respectively. Added 15 mL from each concentration was added to 20 mL of (1 m M) AgNO3 aqueous solution mixed and stirred well for 1 min at 60°C. To start the reduction of Ag ions, the pH adjusted to 8.55, 9, 9.5, 10.5 using NaOH then 300 μL from each colloid solution transferred in each well in 96 micro-plates at different intervals, read by spectrophotometer ELISA-reader at different wavelengths, 340,405, 450,490 and 630 nm as described by (Zeedan, et al., 2018).
Production of silver nanoparticles using natural local Egyptian honey
Zinc oxide and silver nanoparticles determined shape and size by transmission electron microscopy (TEM), each sample diluted with deionized water and 50 μl of each suspension was placed on a formvar-coated copper grid for TEM. For determination concentration, transferred of 300 μL from each colloid solution in 96 micro-plates at different intervals, read by spectrophotometer 96 well ELISA reader at different wavelengths, 340,405, 450,490 and 630 nm.
Characterization of synthesized green zinc oxide and silver nanoparticles
Zinc oxide and silver nanoparticles determined shape and size by transmission electron microscopy (TEM), each sample diluted with deionized water and 50 μl of each suspension was placed on a formvar-coated copper grid for TEM. For determination NPs concentration, transferred of 300 μL from each colloid solution in 96 micro-plates at different intervals, read by spectrophotometer at different wavelengths, 340,405, 450,490 and 630 nm as described by (Zeedan, et al., 2018).
Virus and cells
A local Egyptian strain of BoHV-1 as tissue culture adapted virus used as positive virus control was kindly supplied from Veterinary Research Division, National Research Centre, Cairo, Egypt and Serum and Vaccine Research Institute (VSVRI), Abbasia, Cairo. Madin Darby Bovine Kidney (MDBK) cell line was obtained from Virology Department, Animal Health Institute, Dokki Giza, Egypt.
Virus propagate in tissue cells culture
The tissue cultures infective dose 50 (TCID50) calculated according to (Reed and Munch, 1938) method that was used to determine the viral titer and the anti-viral effect of ZnONPs, AgNPs and Acyclovir.
Cytotoxicity of AgNPs and ZnONPs
The anti-viral effect of AgNPs and ZnONPs on the viability of MDBK cells measured according to (Costa et al., 2018) with some modification. The infected and non-infected MDBK treated with two-fold serial dilutions of each AgNPs and ZnONPs in 2% MEM (0.07 mM in solution as stock solution). Each dilution added in one column of 96 well tissue culture plates 25 µL/well. After 72 h, post incubation, cellular viability measured by Trypan blue stain and the optical density (OD) read at wavelength 450 nm using an ELISA reader.
Inhibitory effect of AgNPs and ZnONPs on BoHV-1
MDBK cells cultured in 96-well plates infected with pre-incubated virus suspension mixed with AgNPs dilution as 25 µL/ well for 2 h. The infected, treated and control MDBK stained with a neutral red stain. The NR stain was prepared as described by (El-Mohamady et al., 2018). and used as 10% in the culture medium (0.33% as stock solution).
Experimental animals
The experiment carried out at the experimental Rabbit Unit of Lab Animal House, Institute at Dokki, Giza, Egypt. Rats and Rabbits housed in separate cages, fed on a balanced commercial ration, and water was available ad libitum, randomly divided into 4 groups then housed in wire cages, lighted for 24 h receiving feeding and water, used in experimental infected with BoHV-1. The animals were bleeding by puncture in the marginal vein in ear after adequate restraint and disinfection of the area. Handling of rabbits and all experimental procedures performed in compliance with the recommendations of the Guide for the Care and Use of Laboratory Animals of the National Research Council (1996).
Experimental design
New Zealand White rabbits 7-8 weeks of age, with a weight of 1-1.5 kg (n-15) divided into 5 groups (each containing 3 rabbits). Group 5: kept as control negative inoculated with 0.25 mL of sterile phosphate buffer saline (PBS) at pH 7.2. Group1 containing 3 rabbits and received intranasal doses of BoHV-1 virus 107 TCID50 /250 μL and Group 2 and 3 were treated with green AgNPs was 30 µg /kg body weight for 5 days post-inoculation, group3: treated with green ZnONPs suspension was 30 µg /kg body weight for 5 days post-inoculation and group4: treated with Acyclovir antiviral drug was 30 µg / kg body weight for 5 days post-inoculation. The animals observed daily for clinical signs or abnormal observation and nasal swabs examined for re- isolation or virus detection with RT-PCR. Blood samples collected from day zero to 3 week post treated at the end of the experiment. The experiment continues for 3 weeks, collected (1 ml / rabbit) into Eppendorf tubes with EDTA3 and then stored at -20oC until used.
Virus isolation
Nasal swabs were collected from all experimental rabbits, using a sterile cotton swab. The swab transported to the laboratory in plastic vials with 1 mL of the transport medium (E199 serum-free medium supplemented with penicillin and streptomycin-amphotericin (10 000 U/mL-10 μg/ mL-0.25 μg/mL) and frozen at -70 °C. Subsequently, swabs washed in medium, centrifuged for 20 min at 1500 rpm and the supernatant filtered through a 0.22 µm membrane. Tenfold dilution of the filtrate then put in contact with MDBK cell monolayers previously cultured in 96-well plates. After the incubation at 37 °C for 60 min, 9.5 mL of E199 medium (E199, Capricorn Scientific, Germany) supplemented with 0.5 % foetal calf serum (FBS, Capricorn Scientific, Germany) was added and the cultures were further incubated at 37 °C for 3 days with daily microscopic examination for the appearance of characteristic cytopathic effects (CPE). If CPE was not observed after 3 days, the flasks with the inoculated cell cultures were frozen and thawed three times and passaged for two more times at three day intervals. In case that no CPE was visible after the third passage, the sample was considered negative. Non inoculated cell monolayers were used as cell controls according to standard procedure (Fuchs et al., 1999; Chothe et al., 2018).
Extraction of viral DNA
DNA extracted using the commercially available DNA extraction kit (Qiagen, USA), as indicated in the manufacturer’s protocol. DNA extraction from the samples extracted from harvested homogenate CAM with clear pock lesion and from the supernatant culture of MDBK cell DNA isolated using QIAamp DNA Mini Kit (Qiagen Ltd USA). The DNA concentration and purification measured using spectrophotometer and elution of DNA in 50 uL of elution buffer and stored at -20 for long-term use.
Polymerase chain reaction (PCR)
PCR specific primers of BoHV-1used glycoprotein B (gB) gene as the target gene as protocol using by (Fuchs et al., 1999). To amplify the target sequence primers for BoHV-1 glycoprotein B (gB) (gB1 5’-TAC GAC TCG TTC GCG CTC TC-3’ and gB2 5’-GGT ACG TCT CCA AGC TGC CC-3’) (Operon Biotechnologies GmbH, Germany) primers were used.1 μl (25 pmol) of each primers, 5 μl of the extracted DNA added to 45 μl of PCR mix containing 2.5 U of Taq DNA polymerase, 5 μL of 10X PCR buffer, 1.5 μl of 50 mM MgCl2, 1 μl of 10 mM of dNTPs mix, The reaction run under the following thermal cycling program: Pre-denaturing at 94°C/3 min; denaturing at 94°C/1 min, annealing at 60°C/1min, extension at 72°C/1min repeated (35 cycles) followed by a final extension at 72°C for 5 min. We used negative and positive control reaction. The agarose gel performed according to (Long et al., 2014). The DNA products shown under ultraviolet (UV) light of the PCR products in a 2% agarose with Ethidium bromide (0.3 mg/ ml) and used ladder 100 bp molecular weight marker.
Real– time PCR
Real–time PCR was performed using a pair of gB sequence specific primers for amplification of target BoHV-1-DNA and a 5’–nuclease oligo probe for the detection of amplified products Real–Time PCR gB–F-TGTGGACCTAAACCTCACGGT, gB–R-GTAGTCGAGCAGACCCGTGTC, FAM–AGGACCGCGAGTTCTTGCCGC–T. 5-carboxyfluorescein (FAM) at the 5’ end, and the acceptor/quencher 6-carboxytetramethylrhodamine (TAMRA) at the 3’ end. This real-time PCR assay is designed to detect viral DNA of all BoHV-1 strains. PCR assay was detected BoHV-1- DNA from experimentally infected rabbits and positive BoHV-1.
Real-time polymerase chain reaction assay
The real-time PCR described in our study used a pair of sequence-specific primers for amplification of target DNA and a 5-nuclease oligoprobe for the detection of amplified products. The real-time PCR reaction was conducted in accordance with (OIE Manual, 2018) with minor modifications, in a Bio-Rad iCycler iQ and the Ct of amplification was determined by data analysis software provided with the instrument. BoHV-1 was propagated in Madin-Darby bovine kidney (MDBK) monolayer cell lines. Serial tenfold dilutions of the virus culture supernatant were prepared from 10 5.68TCID50/50 μl to 10 68TCID50/50 μl. Viral DNA was extracted from each dilution using a Qia Blood mini kit, in accordance with the manufacturer’s instructions. The real-time PCR reaction was performed in duplicate, using eluted DNA and the Ct value was calculated.
Statically analysis
Data presented as mean ± SD and analyzed using a one-way analysis of variance (ANOVA) by using the SAS 9.1 software package (SAS, Cary, NC, USA, significance at P < .05.
RESULTS
Properties of synthesized AgNPs and ZnONPs
UV–Spectrophotometer of prepared silver nanoparticles
The results showed two absorption peaks at wave- length about 340 and 630 nm were were attribute to the formation of AgNPs or ZnONPs.
The cell viability results with different AgNPs and ZnONPs concentrations (µg/mL)
Ag-Ps and ZnONPs were mild cytotoxic effect on MDBK cell culture at 10 to 50 µg /mL concentration but they had cellular toxicity with different degrees with higher concentration more than 60 µg /mL as in Table 2 demonstrated that the cytotoxic effects of different concentration from silver, zinc oxide nanoparticles and Acyclovir on MDBK cells ranged from 60 to 900 µg /mL.
Antiviral Activity of AgNPs, ZnONPs and Acyclovir against BoHV-1 propagated in MDBK cell culture
Complete sheet of MDBK cells tissue culture (four wells for each concentration) were growing in the present of medium 199 containing newborn fetal bovine serum (FBS), contained Penicillin, Streptomycin and Amphotericin. MDBK cells inoculated with BoHV-1 and then Incubated for 1h at room temperature for virus adsorption and then treated with antiviral agents AgNPs, ZnONPs and acyclovir with different concentrations. Plaque Forming Unit (PFU) used for virus titration. Results of virus inhibition determined as the percentage reduction change in OD and calculated as follows= 1-[OD t/ODs] x 100 as in Table 2.

Figure 1: A: showed that the formation of AgNPs in colloid with different honey concentration and different PH 8.5 to 10.5. B: micrograph TEM of silver nanoparticles was demonstrated (spherical particles with particle size diameter < 50 nm. C: TEM micrograph of zinc oxide nanoparticles which showing prismatic shaped while others cubic shaped. The green silver and ZnONPs showed particle size ranged between 20 and 50 nm with the average particle size of 10–50 nm, respectively.
The antiviral effects of green AgNPs and ZnONPs different concentration on MDBK cell culture were evaluated by plaque forming assay (PFU/ml). BoHV-1 incubated with different sizes and different concentrations of Ag NPs at 60 minutes after cell culture inoculating with BoHV-1 were showed dose-dependent virus inactivation as shown in Table 2 and Figure 3. They were causes less virus inhibitory effect in less concentration than 10 ug/ ml (p ≤ 0.001). Of all tested AgNPs and ZnONPs showed the highest antiviral activity already at 10-50 µg/ml in comparison to titer of BoHV-1 infected cultures (6.8 ± 0.076 and 7 ± 0.045 respectively). In comparison to ZnONPs, similar inhibitory effect for AgNPs was achieved at 10- 50 µg/ml (p≤0.001) titer of BoHV-1 decreased from (7 ± 0.089 to 1.75 ± 0.012) for Ag NPs, 100% inhibition was observed at the concentration of 50 µg/ml. The lowest reduction of plaque formation was found for ZnONPs less than 25 µg/ml approximately 20% inhibition. The CC50/TCID50 ratio, selectivity index (SI), showed that the most advantage index was obtained for 25 and 50 µg/ml, they reduced BoHV-1 from 7 to 2.5 and 1.5 respectively.
Nanoparticles protect infected rabbits with BoHV-1
We monitored the experimental infected rabbits with BoHV-1 for 7 at 28 days successive days. Clinical signs and changing in behavior of rabbits, such as lack of appetite, respiratory or changing body temperature and weight loss observed. The BoHV-1 re-detected by conventional PCR and RT-PCR from all treated and infected rabbit groups for determining the antiviral efficiency of silver and zinc oxide nanoparticles. As shown Figure 4 and Figure 5, Treated groups silver, zinc oxide nanoparticles and acyclovir at dosages of 50 ug/ kg/day compared to untreated BoHV-1 infected rabbits group which showed severe clinical sign 14th days post-inoculation but no difference in clinical signs between all treated groups, nanoparticles treated rabbits progress time course of clinical signs observed. The Re-detection of BoHV-1 from 7th to 28th dpi to confirm the protective efficacy of nanoparticles against BoHV-1-infection in vivo by PCR and RT-PCR. Re-detection of nanoparticles treated groups with 50 ug /kg/day by PCR and RT-PCR. The treatment with nanoparticles (5oug/kg/day) showed no difference with the Acyclovir group. Taken together, the results suggest that nanoparticles and Acyclovir could protect rabbits from BoHV-1 infection.
DISCUSSION
Nanoparticles catch the attention of scientific because they have broad range antimicrobial effects (Long et al., 2014). The unique green NPs properties of green synthesized are preferred than chemical and physical methods (Hussain et al., 2016). However, the uses of toxic solvents generated
Table 1: Determination cytotoxicity of zinc oxide and silver nanoparticles concentrations (µg/mL) on MDBK cells by ELISA.
Antiviral agent | Cytotoxic Effect of Green NPs on MDBK cells measured by ELISA Reader = Means ODs ± SD | |||||||||
D 900 ug/ml |
D 450 ug/ml |
D 225 ug/ml |
D 112.5 ug/ml |
D 56.25 ug/ml |
D 28.125 ug/ml |
D 14.625 ug/ml |
D 7.312 ug/ml |
D 3.656 ug/ml |
D 1.828 ug/ml |
|
AgNPs | 0.076± 0.086 |
0.088± 0.023 |
0.166± 0.024 |
0.178± 0.054 |
0.225± 0.065 |
0.197± 0.087 |
0.299± 0.087 |
0.32± 0.043 |
0.35± 0.023 |
0.456± 0.043 |
ZnONPs | 0.065± 0.036 |
0.096± 0.016 |
0.134± 0.085 |
0.156± 0.018 |
0.201± 0.098 |
0.167± 0.098 |
0.267± 0.089 |
0.289± 0.019 |
0.289± 0.098 |
0.489± 0.065 |
Acyclovir | 0.056± 0.016 |
0.098± 0.08 |
0.156± 0.09 |
0.164± 0.018 |
0.265± 0.019 |
0.174± 0.076 |
0.234± 0.016 |
0.281± 0.017 |
0.256± 0.097 |
0.467± 0.076 |
Cell control | 0.476± 0.017 |
0.469± 0.079 |
0.448± 0.075 |
0.342± 0.098 |
0.376± 0.076 |
0.469± 0.046 |
0.448± 0.014 |
0.392± 0.013 |
0.406± 0.023 |
0.406± 0.076 |
Means ODs ± SD: Means of optical density ± Slandered Division; AgNPs: Silver Nanoparticles; ZnONPs: Zinic Oxide Nanoparticles; D: dilution. Significantly different (t≤ 0.005).
Table 2: Effect of different concentrations of Nanoparticles and Antiviral drug on BoHV-1 titer.
NPs | Reduction of BoHV-1 titer from 7 log TCID50 /ml by different NPs concentration | |||||
D 50 ug/ml | D 25 ug/ml | D 12.5 ug/ml | D 6.25 ug/ml | D 3.125 ug/ml | D 1.5625 ug/ml | |
AgNPs + BoHV-1 | 1.5 ± 0.023 | 2.5 ± 0.13 | 6.1 ± 0.006 | 6.5 ± 0.009 | 6.9 ± 0.0034 | 7 ± 0.065 |
Zn ONPs + BoHV-1 | 1.75 ± 0.012 | 3 ± 0.098 | 5.9 ± 0.089 | 6.3 ± 0.029 | 7 ± 0.089 | 7 ± 0.087 |
Acylovir+ BoHV-1 | 1.2 ± 0.062 | 2.4 ± 0.046 | 4.9 ± 0.033 | 5.3 ± 0.034 | 6.2 ± 0.042 | 6.8 ± 0.017 |
BoHV-1 | 6.9 ± 0.098 | 6.8 ± 0.076 | 7 ± 0.056 | 7 ± 0.097 | 6.9 ± 0.034 | 7 ± 0.045 |
Cell control | 0.00 ± 0.00 | 0.00 ± 0.00 | 0.00 ± 0.00 | 0.00 ± 0.00 | 0.00 ± 0.00 | 0.00 ± 0.00 |
Means of BoHV-1 titer ± SD: Means of optical density ± Slandered Division; AgNPs: Silver Nanoparticles; ZnONPs: Zinic Oxide Nanoparticles; D: dilution. No significantly different at (t ≤ 0.005).

Figure 2: Showed that the spectrophotometer absorption wavelength value of AgNPs colloids for different honey concentrations gave a peak at wavelength level 405 and 450 nm. I: 30g at differences pH values at 8.55, 9, 9.55 and 10. II: 40 g honey concentrations at differences pH values. III: showed that the comparing results for different honeys concentrations at different pH values revealed nearly similar peak at wavelengths 405 and 450 nm for all honey groups. IV: showed that the comparing results for different green ZnONPs suspension at different pH values revealed nearly similar peak at wavelengths 405 and 450 nm for all olive leaves plant extracts.
many hazardous problems which harming human and the environment (Viswanath and Kim, 2016; Pandiarajan and Krishnan, 2017). The particles produced by green methods differ than those producing by physicochemical that using expensive chemical reducing agent while, natural product or plant extract are biogenic reduction of metal precursors to corresponding NPs is eco-friendly, (Anbu et al., 2015; Sasidharan et al., 2019). The green NPs were synthesized using olive leaves extract and/or honey initially, the formation of NPs confirmed by changing the mixture color to brownish color after 2-3 hrs from incubation at 37 C and NP formation was confirmed TEM and UV- Spectrophotometer as in Figure 1 Similar finding was made by (Sintubin et al., 2011; Haghighi et al., 2016; Rai et al., 2018). Biogenic materials reduction takes place either in vitro or in vivo by enzymes, sugars, proteins and phytochemicals, like flavonoids, phenolic, terpenoid, mainly act as stabilizing and reducing agents (Kulkarni and Muddapur, 2014; Haider and Kang, 2015).
The present study showed the formation of spherical and prismatic nanoparticles with size diameter ranged between 10-50 nm estimated using TEM. The plant extracts or natural product honey. NPs interact with biomolecules and thus increase the antimicrobial activity by improving the interactions with microorganisms. AgNPs showed 20 times higher antimicrobial activity (Iravani et al., 2019). The plant extracts were produce NPs is based on the added value of the biological material itself. The ZnONPs and the AgNPs nanostructures have a particle size of around 10 to 50 nm and showed a good inhibition effect to the various pathogens (Kulkarni and Muddapur, 2014; Haghighi et al., 2016; Rai et al., 2018; Iravani et al., 2019). The particl sizes of NPs depend on the production methods also, particls shaped strongly depend on pH, temperature, salt concentration and exposure time as shown Figure 1A, B and C and Figure 2 and this finding is agree with (Kawata et al., 2009; Nasrollahzadeh et al., 2019). In the biological method, plant extracts used for synthesis of several metallic nanoparticles. Green synthesis of ZnONPs and AgNPs showed enhanced antibacterial activities against different bacterial pathogens as well as utilization in cancer treatment and diagnostic applications (Jeeva et al., 2014). The green synthesis of ZnONPs and AgNPs are eco-friendly, non-toxic and safe biosynthetic process (Jayaprakash et al., 2017; Kawata et al., 2009). Synthesis of Zn O NPs using water olive leaves extracts and AgNPs used local Egyptian honey as a simple, low cost and reproducible method as shown in Figure 1A, and this result is agree with (Nath and Banerjee, 2013). TEM image confirms the formation of AgNPs and ZnONPs have average size about 20-50 ± 10 nm, which is very similar to previous studies as in Figure 1B and C this results are agreed with (Jamkhande et al., 2019). Natural biological materials are used for in vitro synthetization of NPs, which is useful in purification and bio-reducing agents which mixing with aqueous solution metal precursor under controlled manner. The reaction occurs spontaneously at room temperature but sometimes heating and stirring are used by (Zeedan et al., 2018; Kuotsu et al., 2019; Jamkhande et al., 2019). Each method has advantages and disadvantages, adopted for synthesis method. AgNPs and ZnONPs found in spherical, rod and triangular shape, (Samiei et al., 2016; Dahoumane et al., 2017). ZnONPs and AgNPs exhibited cytotoxic effect with significant depletion of superoxide dismutase (SOD and reactive oxygen species (ROS) levels as shown in Tables 1 and 2, Figure 3A and 3B. Our results demonstrated that ZnONPs are exert cytotoxicity more than AgNPs at same concentrations but they are dose- and time-dependent effects. While Ag NPs showed mild effect on viability of cells as shown in Figure 3A and 3B and Tables 1 and 2 compared to control group, inhibitory effect on BoHV-1 induced by ZnONPs from concentration ranged between 10 to 50 μg/mL which highly significantly at (p < 0.01). Although, AgNPs showed slightly depletion of MDBK cells but it induced more inhibitory effect on BoHV-1. While, the cells exposed to ZnONPs produced more cytopathic effect than AgNPs. Our results revealed that the ZnONPs had significant toxicity than AgNPs which had slight toxic as superoxide dismutase is an antioxidant enzyme, although, the main mechanism of NPs cytotoxicity may differ, depending on the types of NPs, on the other hand the ZnONPs were more toxic than AgNPs in MDBK cells.

Figure 3: A: Normal MDBK cell culture non- infected or non-treated with nanoparticles, B: inoculated MDBK cell culture with suspected BoHV-1 virus 10 7 TCID 50% which showing cell rounding, cells aggregations and formation clusters of rounded cells cytopathic effect (CPE) stained with Hematoxylin and Eosin, (Magnification power 100X).
ZnO and AgNPs exhibited inhibitory effect on BoHV-1 post tissue culture infection but cytopathic effect occurred on infected MDBK with BoHV-1. The mechanism of ZnONPs and AgNPs to induce inhibitory effects on BoHV-1 is due to small size of nanoparticles more effective against BoHV-1 as in Table 2 (Kawata et al., 2009). ZnONPs and AgNPs stuck to the Sulphur in the gp120 glycoprotein knobs that inhibiting the normal activities of the virus, which alter normal viral functions.
Our study, ZnONPs and AgNPs protected the MDBK cell culture from BoHV-1 infection at a concentration of 20-50±10 nm as shown in Table 2 and Figure 3 and this results is agree with the finding recorded by (Chandra et al., 1999). El-Mohamady et al. (2018) who reported that ZnONPs and AgNPs inhibit poliovirus and showed the nontoxic effect of the cell culture even at concentrations up to 100 ppm. ZnONPs and AgNPs can control the CPE of MDCK cells caused by BoHV-1. ZnONPs and AgNPs get stuck to the outer proteins of the virus sequentially inhibiting the normal function of the virus (Chandra et al., 1999).
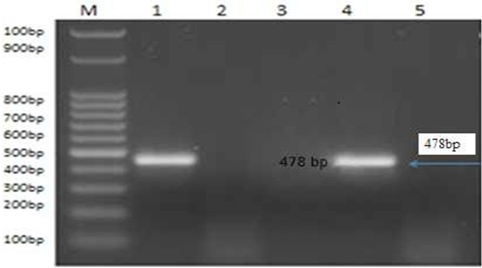
Figure 4: PCR product of the 478bp for amplified of BoHV-1 using primer for GI glycoprotein gene fragment, Lane M: 100bp ladder. Lane 1: control positive BoHV-1. Lanes 2: control negative samples Lanes 3 and 5: treated by AgNPs or ZnONPs inhibitory effect on sBoHV-1 in experimentally infected rabbit from nasal swabs Lane 1: control positive BoHV-1. Lane 4: control positive group.

Figure 5: showed that the real-time PCR baseline subtractive Ct fit view of positive BoHV-1 1 DNA plotted against cycle number from left to right, Ct1 represents reactions containing copy number from nasal swabs of infected rabbit groups without treatment control positive group. No Ct curve denoted good inhibitory NPs effect on BoHV-1.
The present study detection of BoHV‐1 DNA by PCR and real-time PCR assays which satisfactory amplification of 10 copies of target genome (El-Sayed and Kamel, 2018). The standard Ct 1 and 2 generated from the positive BoHV-1-DNA control and control positive infected rabbit group can evaluated by the numbers of copy. Real-time PCR positive result appears a linear correlation between Ct2 values of the positive control virus and tested samples from infected rabbits with BoHV-1 as shown in Figures 4 and 5 and this result is agree with (Winn, 2006). Evaluation of NPs post infected rabbit groups treatment while PCR and real time PCR -positive for positive in control positive infected rabbits not received any treatment as in Figure 4A and 5 and this result is agree with (Biswas et al., 2013; Zeedan et al., 2019) which explain PCR negative results for virus could be due to antiviral effect of acyclovir treated rabbit groups or NPs inactivated BoHV-1. Unlike virus isolation, BoHV-1 detection by real-time PCR not only depends on the amount of infectious virus particles but also detects inactivated virus in the sample (Kuotsu et al., 2019). The amount of virus shedding in the nasal secretion may vary from group to group. Detection of NPs antiviral effects on experimentally infected rabbits. However, no difference between RT-PCR and PCR values were recorded or in positive BoHV-1 control and untreated group. The highest efficacy of green AgNPs followed by ZnONPs gives a new hope for finding new antiviral agents against BoHV-1, and their mechanism against latent BoHV-1 should be further investigated to develop more efficient antiviral therapeutics.
CONCLUSIONS
In the present study zinc oxide and silver nanoparticle green synthesis by olive leaves extract and Egyptian honey. In vitro, green silver nanoparticles induced mild or little toxicity on MDBK cell culture at concentrations ranged from 25 to 50 ug/ ml. The green zinc oxide and silver nanoparticles could control and inhibiting BoHV-1 inactivity titer and protected rabbits against experimental infections with BoHV-1. The highest efficacy of green AgNPs followed by ZnONPs gives a new hope for finding a new antiviral agent against BoHV-1, and their mechanism against latent BoHV-1 to develop more efficient antiviral agent.
ACKNOWLEDGMENTS
This research was funded by National Research Centre, Dokki, Egypt, Research Project ID Number: # 12010138 #.
Authors Contribution
Zeedan, GSG found research idea, planned the study design, performed data, performed the laboratory work performed the laboratory work PCR, RT-PCR and drafted the manuscript. EL-Razik KAA Allam AM and Abdalhamed AM all shared in the research’s idea, sharing shared designed work , collection of blood samples during in experiment and helped in manuscript preparation. Zeina HAAA interpreted the data results and helped in manuscript preparation. All authors read and approved the final manuscript.
Conflict of interest
The authors of the present work declare that is no conflict of interest in this work.
REFERENCES