Advances in Animal and Veterinary Sciences
Research Article
Biosynthesis and Characterization of Silver Nanoparticles by Silybum marianum (silymarin) Fruit Extract
Zahraa M. Ayad, Orooba Mohammed Saeed Ibrahim*, Luma W. Omar
Department of Physiology and Pharmacology, College of Veterinary Medicine, University of Baghdad, Iraq.
Abstract | This study was carried out to synthesis of silver nanoparticles (AgNPs) by using Silybummarianum fruit extract which is very simple and eco-friendly method. The separation of this nanoparticle was performed by centrifugation while the identification was by UV-Visible spectroscopy, X-ray diffraction, Fourier Transmission Infrared Spectroscopy (FTIR) and Scanning Electron Microscopy (SEM) methods. Reduction of the Ag+ to Ag0 during exposure to the S. marianum fruit extract was followed by color change of the solution from colorless, yellow to dark brown within 24 hours. It is observed that surface Plasmon resonance peaks of the maximum absorbance of silver-nanoparticles occurs at 425 nm, indicating that AgNPs were produced. Involvement of the flavonoids group (flavolignans) in the synthesized AgNPs was manifested from the result of the FTIR. Particle size was recorded according to the data exhibited from the XRD results at 2θ around 25 nm which was calculated by using the Dubai-Scherrer equation. The silver nanoparticles synthesized by the help of silymarin fruit extract were scanned using SEM. From the SEM image reveals that the silver nanoparticle seems to be spherical in shape. From the results of the current study concluded that silymarin fruit extract could be considered as a good source for synthesis of stable AgNPs in short time, and the process of synthesis was simple, low cost and eco-friendly.
Keywords | Silver, Nanoparticles, Silymarin fruit extract, AgNPs , Eco-friendly.
Editor | Kuldeep Dhama, Indian Veterinary Research Institute, Uttar Pradesh, India.
Received | October 19, 2018; Accepted | November 11, 2018; Published | December 03, 2018
*Correspondence | Orooba Mohammed Saeed Ibrahim, Department of Physiology and Pharmacology, College of Veterinary Medicine, University of Baghdad, Iraq; Email: doroobam2000@gmail.com
Citation | Biosynthesis and characterization of silver nanoparticles by silybum marianum (silymarin) fruit extract. Adv. Anim. Vet. Sci. 7(2): 122-130.
DOI | http://dx.doi.org/10.17582/journal.aavs/2019/7.2.122.130
ISSN (Online) | 2307-8316; ISSN (Print) | 2309-3331
Copyright © 2019 Ayad et al. This is an open access article distributed under the Creative Commons Attribution License, which permits unrestricted use, distribution, and reproduction in any medium, provided the original work is properly cited.
INTRODUCTION
‘’nano’’ is derived from a Greek word meaning dwarf or extremely small (Fakruddin et al., 2012).Nanotechnology is one of the key technologies of the 21st century. Nanotechnology have various applications such as in agriculture, Environmental chemistry, Food science, Veterinary medicine, Marine, Medicine detection (Cardiac therapy, Dental care, Diagnostic techniques, Skin therapy and Cancer therapy) and Tissue engineering, Industries and many others (Jean Buzby, 2010). Silver nanoparticles (AgNPs) have received essential attention due to their chemical, attractive electronic and optical properties (Austin et al., 2014). AgNPs have been synthesized by different physiochemical techniques such as chemical reduction (Khan et al., 2011).These methods have effective yield, but they are associated with the limitations like use of toxic chemicals and high operational cost and energy needs. Considering the drawbacks of physiochemical methods, cost-effective and energy efficient new alternative for AgNP synthesis using microorganisms (Sharma et al., 2009), plant extracts (Song et al., 2009) and natural polymers (Huang et al., 2004) as reducing and capping agents are emerging very fast. The association of nanotechnology and green chemistry will unfold the range of biologically and cytologically compatible metallic nanoparticles (Philip 2010; Srikar et al., 2016). Silymarin is a complex mixture of polyphenolic molecules, including seven closely related flavonolignans (silybin A, silybin B, isosilybin A, isosilybin B, silychristin, isosilychristin, silydianin) and one flavonoid (taxifolin) (Kroll et al., 2007).The main mechanism considered for the synthesis of nanoparticles mediated by the plants is due to the presence of phytochemicals. The major phytochemicals” responsible for the spontaneous reduction of ions are flavonoids, terpenoids, carboxylic acids, quinones, aldehydes, ketones and amides (Prabhu et al., 2012).According to pharmacological studies, silymarin has been accepted as a safe herbal product, since using the physiological doses of silymarin is not toxic unless the improper administration of therapeutic dosages (WenWu et al., 2009; Ramakrishnan et al., 2009; Toklu et al., 2007). Milk-thistle plant (Silybum marianum) extract is used for production of AgNPs through the Keto-enol Tautomerization. Therefore this study aimed to biosynthesis and characterization of AgNPs by milk thistle fruit (silymarin) extract and characterization of AgNPs by UV-visible spectroscopy, XRD, FT-IR, SEM.
MATERIALS AND METHODS
Preparation of the Silymarin Extract
Silymarin (Milk thistle fruit) plant was purchased from (MADAUS)® GmbH Pharmaceuticals, Germany, and it is pale yellow powder. The aqueous extraction of the silymarin fruit was prepared by adding 5g. of fruit powder to 100ml of boiling deionized water (DI). After boiling for 20 min, the extract was filtered with What man No.1 filter paper (Mohammadinejad et al., 2013).
Biosynthesis of Silver Nanoparticles
The synthesis of AgNPs was made by mixing 47 ml of 1mM Silver nitrate (AgNO3) solution with 3 ml of milk thistle fruit extract with stirring and leave at room temperature for formation of AgNPs. The changing of solution color was measured at every one hour until 24 hours. The changing in the color intensity after the reduction of Ag+ to AgNPs by Silymarin plants fruit extracts with increasing time of reaction was recorded (Mohammadinejad et al., 2013; Nagati et al., 2012).
Centerifugation
Silver nanoparticles solution was centrifuged at 15000 rpm for 30 minutes. The pellets were washed 3 times with 20 ml of distilled water, and finally dried at 60°C, to dispose of the free proteins/catalysts that are not topping the AgNPs. The centrifugation process was done at time 1-24hrs. This was done in order to know the effect of time incubation on amount of AgNPs synthesized (Awwad et al., 2012).
Separation and Identification of Silver Nanoparticles (AgNPs)
This work was done in the Ministry of Science and Technology Laboratories / Department of Materials Research.
Spectrophotometery: The stability and formation of AgNPs was monitored with ultraviolet visible spectrophotometer apparatus in wavelength ranged from (300-600) nm. For the UV-Vis spectral analysis. Absorbance of this solution was measured at one hr interval for 1-24hrs and the changing in the color was observed gradually as it turned deep brown at the end of 24hrs (Awwad et al., 2013).
X-Ray Diffraction (XRD): Solution of the developed AgNPs was centrifuged at 10,000 rpm for 30 min. The solid residues of Ag NPs were washed twice with deionized distilled water and then dried at 80ºC to obtain powder AgNPs used for X-ray powder diffraction measurements. The powder X-ray diffraction (XRD) patterns were recorded on (Shimadzu XRD-6000) with copper radiation (Cu Kα, 1.5406 Å) at 40 kV and 30 mA (Bykkam et al., 2015).
Fourier Transform Infrared Spectroscopy (FT-IR): FT-IR measurements were carried out using (FT-IR Spectroscopy, ABB-Specro-Lab-MB3000, UK) the range from 3500 cm-1 to 500 cm-1. After complete reduction of AgNO3 ions by silymarin fruit extract, the mixture was centrifuged at 10000 rpm for 10 min to remove protein or other bioorganic compounds that were present in the solution. The silver nanoparticles pellet obtained was air dried. The dried nanoparticles were mixed with the potassium bromide (KBr) to made thin pellets and were used for FT-IR analysis in transmittance mode (Kalaiarasi et al., 2013).
Scanning electron microscopic analysis: There were no reported differences in the shapes and size of AgNPs at various initial biomaterial concentrations (Huang et al., 2007), therefore the samples for electron microscopy were prepared from the extract and various time of reactions. The biomasses had settled in the base of the cone like carafes and the suspension over the accelerate was tested for scanning electron microscopy (SEM) perception. Scanning electron microscopy of the aqueous solution samples of AgNPs prepared by setting a one drop of the solution on the carbon-covered copper grids and the films on the SEM system permitted standing for two minutes, after that there is removing of the extra solution by using a blotting paper and drying the grid. The size appropriation of the subsequent nanoparticles evaluated on the basis of SEM micrographs (Srinivas et al., 2013).
ReSULTS
Visual Observation
A quick and traceable change was observed in bio reduction medium after addition of silver nitrate into S.marianum fruit extract. In order to control the particle size of AgNPs, 1 mM AgNO3 was added to the aqueous fruit extract and incubated at 65°C at pH 8.0. Synthesis was confirmed by visual observation of the fruit extract and AgNO3. The mixture of fruit extract and AgNO3 showed a color change with time from colorless to pale yellow after 30 minutes to deep brown color after 24hr. No color change was observed during incubation of fruit extract without AgNO3 (Figure 1). The appearance of a brown color in AgNO3-treated fruit extract suggested the formation of AgNPs.
B. Centrifugation
The centrifugation of AgNPs solution at different incubation time interval (1-24hrs), the samples were dried and weighing after washed three times with 20 ml of de-ionized water to get rid of the free proteins/ enzymes that are not capping the AgNPs. The changes in pellet weight (gm/time) gave an evidence of correlation between incubation time and the amount of synthesized AgNPs. After 1, 2 and 3 hrs of incubation time, there was a decrease in pellet weight (0.15, 0.19 and 0.2 gm) respectively, when they were compared with 12, 18, and 24 hrs of incubation time (0.35, 0.42 and 0.55 gm). Three ml of S. marianum fruit extract mixing with 47 ml of 1mM AgNO3 solution, gave a deep dark color pellet.
Separation and Identification of Silver Nanoparticles
UV–visible absorbance analysis: The formation of AgNPs was monitored with color change and UV-Vis spectrum. The reduction of silver ions into AgNPs started after 30 minutes of reaction and completed after almost 24 hours. The absorption spectra of AgNPs solution consists a single sharp surface plasmon resonance band at 425 nm (Figure 2A). The most characteristic part of silver solution is a narrow plasmon absorption band observable in the 300 – 600 nm regions. The distinct visible peak was observed at wave length 425 nm which is an indication of reduction of silver. This indicates that by UV method, silver gets reducing in a faster way than the conventional method. (Figure 2B) shows the UV–vis absorption spectra of silver synthesized nanoparticles after storage for 8 months to check the stability of the AgNPs As it can be seen, the absorption peaks of the AgNPs shift only slightly, without a significant change in the color.
X-Ray Diffraction (XRD): XRD is a rapid analytical technique used for characterization and identification of a crystalline material of synthesized nanoparticles and can provide information on unit cell dimensions. The X-ray diffraction pattern of the biosynthesized silver nanostructure was demonstrated and confirmed by the characteristic peaks observed in the XRD image (Figure 3).
The XRD pattern showed seven intense peaks (27.56°, 29.80°, 32.90°, 46.82°, 55.05°, 57.81°, 77.60°) in the whole spectrum of 2θ value ranging from 20 to 100 and indicated that the structure of silver nanoparticles is face centered cubic (fcc). These patterns corresponding to (122), (111), (200), (220) and (311) respectively. The set of lattice planes were observed and compared with the reference values of Joint Committee on Powder Diffraction Standards (JCPDS: 89-3722). The average particle size of the silver nanoparticles formed in the biosynthesis process was determined using Scherr’s equation and was estimated as ( 25.26) nm.
Fourier Transform Infrared Spectroscopy (FT-IR): FTIR analysis was conducted, in order to determine the functional groups between the reducing agent (silymarin fruit extract) and AgNO3 and predict their role in the synthesis of silver Nanoparticles. And all these functional groups was shown as a peaks in the FTIR Spectra in the (Figure 4). The study showed obvious absorption bands located at 3328, 2936, 2360, 1640, 1384, 1088, 1024, 928, 888, 832,720,672,600cm-1. The absorption bands 3328 mean N–H stretch, also may be belongs to the stretching vibration of intermolecular hydrogen bonded O–H group in alcohols and phenols, 2936 assigned to aldehydic C–H stretching, and the bands at 2360 cm−1 was attributed to carbon dioxide. While the bands 1640, 1384, 1088, 1024 mean С–O or N–H, C=O, C–N stretching , C-O group stretching, respectively. In addition to the bands 928, 888, 832 cm-1 assigned to C=CH2 , and the bands near 720,672,600cm-1 means C–H bending vibrations and amine groups (–NH).
Scanning electron microscopic analysis (SEM): Scanning electron microscopy (SEM) was used to visualize the morphology (shape and size) of nanoparticles. The SEM images of the prepared AgNPs are shown in the (Figure 5). From the different magnification of SEM image of AgNPs reveal that the particles are agglomerated, and almost the AgNPs particles appear spherical in shape with mean average diameter of 25.26nm.
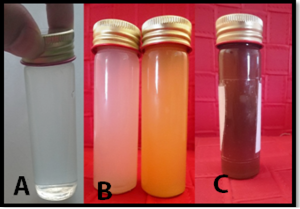
Figure 1: Color Change during synthesis of Silver Nanoparticles, A- AgNO3 only, B- white color when mixing of silymarin fruit extract with AgNO3 immediately then change to yellowcolor after 30 min. C- Deep brown color at 65 Ϲᵒ within 24hrs. of reaction time.
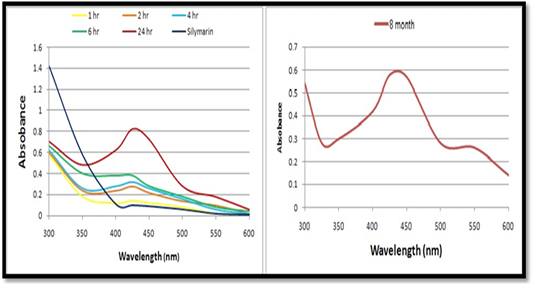
Figure 2: A-UV–visible absorption spectra of synthesized AgNPs by silymarin fruit extract at different times. B- UV–visible absorption spectra of synthesized AgNPs by silymarin fruit extract after 8 months
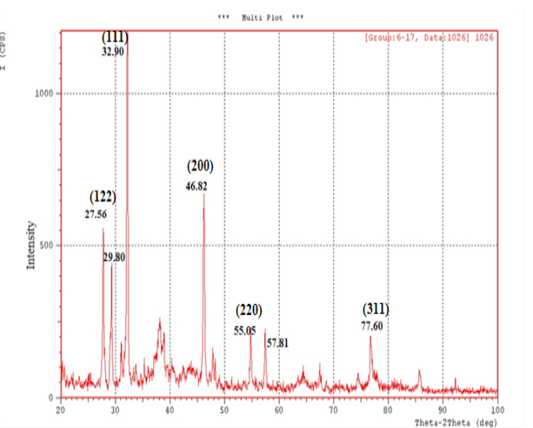
DISCUSSION
Biosynthesis of Silver Nanoparticles by Silymarin Fruit Extracts
Color Change: Biosynthesis of nanoparticles using eco-friendly approach has been the area of focused research in the last decade. Green sources act as both stabilizing and reducing agent for the synthesis of shape and size controlled nanoparticles. AgNPs were synthesized using extract of silymarin fruit. Gradual increase in color of the extract after addition of 1 mM AgNO3 which is considered as an indication of the presence of AgNPs. The appearance of a deep -brown color confirms the existence of silver nanoparticles in the solution. The time duration of change in color was 24 hours. This change in color of the reaction mixture was taken as a primary evidence for the formation of silver nanoparticles. The time duration of change in color and thickness of the color varies from plant to plant. The reason could be that the quantitative variation in the formation of silver nanoparticles (or) availability of H+ ions to reduce the silver. The aqueous silver ions when exposed to herbal extracts reduced in solution, there by leading to the formation of silver hydrosols (Niveditha and Sukirtha, 2018).
The reduction of Ag+ to Ag0 occurs by combinations of biomolecules such as proteins, polysaccharides, and flavonoids (Park et al., 2011; Vahabi et al., 2011; Mansoori, 2013). Certain biological synthesis of metal and their alloy nanoparticles is nontoxic, eco-friendly and a low-cost technology for the large-scale industrial production of well-characterized nanoparticles (Vahabi et al., 2011; Mansoori, 2013). The synthesis of AgNP by biological entities is due to the presence of large number of organic chemical like carbohydrate, fat, proteins, enzymes and coenzymes, phenols flavanoids, terpenoids, alkaloids, gum, etc capable of donating electron for the reduction of Ag+ ions to Ag0. The active ingredient responsible for reduction of Ag+ ions varies depending upon extract used (Jha et al., 2009).
The formation of AgNPs was confirmed by changing in the solution color from pale yellow to dark brown. This result of color changes confirmed by Kora et al. (2010) and Vijayaraghavan et al. (2012) and Rajoriya (2017) when reported similar changes in the color. Also, reported the optimum conditions for the increase yield of synthesis of AgNPs when studied physicochemical parameters, temperature, pH of the medium, concentration of silver nitrate, concentration of plant extract and reaction time. Bio-reduction involves reducing metal ions or metal oxides to 0 valence metal NPs with the help of phytochemicals like polysaccharides, polyphenolic compounds, vitamins, amino acids, alkaloids, terpenoids secreted from the plant (Vigneshwaran et al., 2006; Vigneshwaran et al., 2007).
As well as the whole plant of Silymarum.marianum found to contain the phytochemicals flavonoids, phenols and tannins which act as good bioreductant agent. (Syed Muhammad et al., 2011). The main active constituents of this plant are Flavonolagnan collectively known as silymarin (Lecomte, 1975). This change in the color of the aqueous solution at different period from yellowish to deep brown after mixing due to the excitation of surface plasmon vibrations with the AgNPs (Mulvaney, 1996; Mohammadinejad et al., 2013). It is assumed that less the concentration of the plant extracts and AgNO3 used, the smaller will be the size of the nanoparticles. This hypothesis has been proved by several researchers (Ghosh et al., 2011; Chandran et al., 2014) also may be confirmed again by the current study. The appearance of brown color in the reaction solution was a clear indication of the formation of AgNPs in the reaction mixture (Kumar et al., 2008; Kumari and Philip, 2013).
a. UV–visible absorbance analysis: The absorption spectrum of reaction medium confirmed the presence of AgNPs. The characteristic absorption peaks of synthesized AgNPs recorded between 400 nm and 430 nm indicated the formation of AgNPs. It uses light in the visible and adjacent (near UV and near-infrared (NIR) ranges where molecules may undergo electronic transitions (Jia et al., 2006; Pal et al., 2007; Nazeruddin et al., 2014). Intensity of color increased is directly proportional to the reaction time. Also it was observed that there is no correlation always between the color development and the increase in absorbance exhibited by the nanometal synthesized (Leela and Vivekanandan, 2008).
AgNPs exhibit yellowish brown colour in water and this color arises may be due to the excitation of surface plasmon resonance (SPR) effect and reduction of AgNO3 (Noginov et al., 2007). When a particular wavelength is matched to the size of NPs dipole oscillation is generated in the compensated form of the induce polarization and the electrons in the NPs resonate, introducing a strong absorption (Mulvaney, 1996; Moskovits and Vickova, 2005). Light plays a significant role in the phytosynthesis of AgNPs. Rapid production of AgNPs was noticed under sunlight, may be due to the photosensitization of molecules present in the plant extract, similar findings were reported by Rastogi and Arunachalam, (2011) and Rajoriya et al. (2016) It has been observed that light is significantly executing the green synthesis of AgNPs and the effect may vary species to species. Temperature is another physical factor which play a vital role in controlling the nucleation reaction of NPs fabrication. Better synthesis of AgNPs at 65°C was recorded.
The increase in temperature increased the rate of formation of AgNPs from silver ions, retarding the secondary reduction process. Our results are in good agreement with Song et al. (2009) and Kaviya et al. (2011). Increase in absorbance of reaction mixture with the increase in incubation temperature markedly illustrates the higher productivity of AgNPs, at elevated temperature (Van Hyning et al., 2001; Dubey et al., 2010b). The sharpness in absorbance peak depends on size of the synthesized NPs as with higher temperature, particle size may be smaller, which results in the sharpness of the plasmon resonance band of AgNPs.pH is also one of the key influencing factor possibly responsible for biosynthesis of AgNPs. Therefore, pH 8 is suitable for AgNPs synthesis. Vanaja et al. (2013); Thamer and Almashhedy (2014) and Rajoriya (2017) also reported that AgNPs show maximum stability at the pH 8.2 and pH 9.
It is well known that AgNPs possess characteristic absorbance peak between 400 nm to 450 nm. If the peak raised above this range (400 nm to 450 nm), causes aggregation or precipitation resulting particle with large size while if the peak shift below this range (400 nm to 450 nm) indicate that the nano solution contains other participants (impurities, organic species, solvent etc.). The shift in absorption peak may be highly indicative to size, morphology, quantity and NPs growth. The difference in the morphology of the NPs synthesised may be the possible reason for the difference in optical properties (Xu and Kall, 2002).The stability of synthesized silver nanoparticles after storage for 8 months. As it can be seen, the absorption peaks of the AgNPs shift only slightly, without a significant change in the color. This indicates that the prepared AgNPs are stable over a long period (Liu et al. 2009; Mohammadinejad et al., 2013).
b. X- Ray Diffraction (XRD) analysis:
In the present study, the XRD analysis was an effective characterization to confirm that the synthesized silver nanoparticles was obviously crystalline in nature. (Yallappa et al., 2015). XRD is a useful tool in obtaining information about the atomic structure of materials. XRD is not only usually used for qualitative identification of minerals in geological samples by fingerprinting approach but also is used for the quantification of mineralogical data (Al-Jaroudi et al., 2007). XRD is a valuable characterization tool to prove the formation of AgNPs, determine the crystal structure and calculate the crystalline nanoparticle size (Philip, 2010; Chanda, 2013).
The occurrence of sharp peaks in the XRD pattern was due to high degree of crystallinity of silver nanoparticle and the presence of organic compounds in the plants such as flavonoids , phenols which responsible for reduction and stabilization of silver ions in the produced AgNPs (Roopan et al., 2013). The diffraction peaks were broad indicating that the crystallite size is very small. Also, the XRD result was in agreement with the other earlier researches (Kumar et al., 2014; Sivakumar et al., 2011). Therefore silymarin fruit extract can be considered as promising source of development of AgNPs.
c. Fourier Transform Infrared Spectroscopy (FT-IR) analysis: FT-IR analyses were accomplished to identify the potential biomolecules responsible for the capping and stabilization of silver nanoparticles synthesized by using milk thistle fruit extract. In the present study, different FT-IR spectra are generated that displays strong absorption bands which in turn suggest the presence of the following molecules O–H, N– H in the milk thistle fruit extract which indicate the presence of phenols and flavonoids group. As well as 1640 stretching bands originate from the functional group N– H which indicate the presence of amide І stretch vibrations in the amide linkages of the proteins. The band at 1384 cm-1 corresponds to C=O stretching of aromatic amine, and this could be assumed to the presence of phenolic group. And the bands 720,672,600cm-1 is assigned to bounded amine groups (–NH) of the fruit extract, similar findings were reported by Niveditha and Sukirtha (2018).
The detailed analysis of FTIR spectra revealed that the presence of flavonoids and polyphenols, amide groups of proteins apart from other phytochemicals, which are mainly responsible for the formation of AgNPs by reducing the AgNO3 (Kumar et al., 2010; Mittal et al., 2013). Result suggests that molecules attached with AgNPs have free and bound amide group and the compounds attached with the AgNPs could be polyphenols with aromatic ring and bound amide region. The similarities between the spectra of extracts with their AgNPs with some marginal shifts in peak position, clearly indicate the presence of residual plant extract in the sample as a capping agent to the AgNPs.
The shift in the peaks of the spectrum was observed and the remaining peaks unchanged suggesting the possibility of binding of protein with AgNPs. The proteins could most possibly form a coat covering metal NPs (i.e. capping of AgNPs) for the prevention of agglomeration of the particles and stabilizing in the medium (Basavaraja et al., 2007). The carbonyl group from amino acid residue and proteins has the stronger ability to bind metal indicating that the proteins could possibly form a layer covering the metal NPs (i.e. capping of AgNPs) to present agglomeration thereby stabilize the medium (Huang et al., 2007).
It is well known that biological components interact with metal salts and mediate reduction process with these functional groups (Ajitha et al., 2014). Comparison of the FTIR spectra of AgNPs with C. nobile aqueous extract revealed the presence of functional groups such as amines, carboxylic acids, aldehydes and alcohols which are responsible for reducing and capping the silver ions (Philip, 2011). Since biomolecules are responsible for the reduction and stabilization, the biosynthesized nanoparticles are environmentally benign and nontoxic (Sathishkumar et al., 2011).
d. Scanning electron microscopic analysis (SEM) analysis: SEM is a technique that uses electrons instead of light to form an output image (Klein et al., 2012). The SEM analysis is employed to characterize the size, shape, morphology and distribution of synthesized AgNPs (Chanda, 2013). The SEM micrographs also indicate the purity and polydispersity of resulting AgNPs (Mittal et al., 2013). In order to investigate the morphology and size of the prepared silver nanoparticles, SEM images should be recorded.
The green synthesis of AgNPs from silymarin fruit extract exhibit many shapes and size, but the predominant shape was spherical with diameter around of 25 μm. it is obviously observed that the sample consists of highly agglomerated nanoparticles which have formed large aggregates, it can be observed that larger particles of AgNPs are formed due to aggregation of nanoparticles during sample preparation (Vijaykumar et al., 2014).
CONCLUSION
Silymarin fruit extract was a good source for synthesis of stable AgNPs in short time, and the process of synthesis was simple, low cost and eco-friendly.
ACKNOWLEDGEMENTS
Authors acknowledge to the Department of Physiology and Pharmacology, College of Veterinary Medicine, University of Baghdad, Baghdad, Iraq. Ministry of Science and Technology Laboratories / Department of Materials Research for support.
CONFLICTOF INTERESTS
There is no conflict of interest.
AUTHORS’ CONTRIBUTION
All the authors have contributed equally in terms of giving their technical knowledge to frame the article.
REFERENCES