Advances in Animal and Veterinary Sciences
Research Article
Maximization of Bio-Ethanol Production by Yeasts using Sugar Cane and Sugar Beet Molasses
Zaghloul, R.A.1, Solafa A. Ismail2, Gamal Enan3*, Rasha M. El-Meihy1, Abdel-Rahman H.M.1
1Agricultural Microbiology Department, Faculty of Agriculture, Benha University, Moshtohor, Kaluybia, 13736, Egypt; 2Faculty of Technology and Development, Zagazig University, Egypt; 3Faculty of Science, Zagazig University, Egypt.
Abstract | This research was carried out to evaluate the bio-ethanol production by yeasts. Out of the 7 tested yeast isolates, only 5 isolates were able to produce bio-ethanol efficiently during the fermentation of sugarcane molasses. It is worthily to mention that the highest bio-ethanol producers from sugarcane and sugar beet molasses were Saccharomyces cerevisiae F15 (S. cerevisiae) followed by Candida kefyr EMCC77 (C. kefyr). Therefore, they were selected for further experiments. Regarding the effect of environmental factors on bio-ethanol yield, the optimum pH values for bio-ethanol yield were 4.5 and 5 for S. cerevisiae and C. kefyr respectively. Whereas the optimum incubation temperature for bio-ethanol production for both S. cerevisiae and C. kefyr was 32oC. Maximum production of bio-ethanol by S. cerevisiae and C. kefyr was optimally obtained after 48hours incubation period. Under all optimum conditions, S. cerevisiae gave bio-ethanol yield from sugar cane molasses higher than that obtained by C. kefyr and vice versa in case of sugar beet molasses. Finally, immobilized cell technique showed better results in bio-ethanol production than free cells. In view of the obtained results, it could be recommended that the S. cerevisiae and C. kefyr can be used for industrial production of bio-ethanol.
Keywords | Bio-ethanol, Biofuels, Immobilization, Fermentation, Sugarcane molasses, Sugar beet molasses, Saccharomyces cerevisiae, Candida kefyr EMCC77
Received | July 17, 2021; Accepted | August 22, 2021; Published | October 15, 2021
*Correspondence | Gamal Enan, Faculty of Science, Zagazig University, Egypt; Email: gamalenan@ymail.com
Citation | Zaghloul RA, Solafa AI, Gamal E, Rasha ME-M, Abdel-Rahman HM (2021). Maximization of bio-ethanol production by yeasts using sugar cane and sugar beet molasses. Adv. Anim. Vet. Sci. 9(12): 2069-2076.
DOI | http://dx.doi.org/10.17582/journal.aavs/2021/9.12.2069.2076
ISSN (Online) | 2307-8316; ISSN (Print) | 2309-3331
Copyright © 2021 Zaghloul et al. This is an open access article distributed under the Creative Commons Attribution License, which permits unrestricted use, distribution, and reproduction in any medium, provided the original work is properly cited.
INTRODUCTION
Due to the environmental pollution caused throughout by fossil fuels, it was necessary to find out other eco-friendly renewable and sustainable sources of energy (Demirbas, 2009; Kiran et al., 2014; El-Gazzar et al., 2021). As liquid biofuels represent 40% of renewable energy sources, they are prioritized worldwide (Tan et al., 2008). The use of liquid biofuels helps in reduction of greenhouse gas emissions, creation of job opportunities, regional development and supply security (Demirbas, 2009; Enan et al., 2018; El-Bahr et al., 2021). Biofuels are of certain interest world wide as they donot pollute the environmental. Bio-ethanol is one of the most widely used biofuel for transportation worldwide, since it is a renewable, nontoxic, biodegradable resource and it is oxygenated (Hernández ant Kafarov, 2009; Enan et al., 2020; Osman et al., 2021a). Worldwide, bio-ethanol fuel share about 10–20% of the gasoline consumption by 2030 (Walter et al., 2008).
As reported previously, growth and bio-ethanol production of yeasts are influenced by nutritional and environmental conditions (Saini et al., 2018; Abdel-Shafi et al., 2020; El-Gazzar and Ismail, 2020). Temperature and pH value effect membrane turgidity, enzymatic activity and metabolism of yeast cells (Chohan et al., 2020; El-Sayed et al., 2020). Yeasts prefer acidic pH values that enhance growth at the industrial level (El-Gazzar and Enan, 2020; Osman et al., 2021b). Since, yeasts tolerate high temperature and low pH, they are ideal for bio-ethanol production. Yeasts require different nutrients of which sugar mostly limit their growth and activity. Sugar cane and beet molasses are the most utilized sucrose-containing sources for bio- ethanol production. Molasses and other intermediates from sugar beet processing are very good raw materials for bio-ethanol production as they are used for fermentation without modification (Rodríguez et al., 2010). Molasses is a traditional raw material for distilleries in Egypt, and about 90% of bio-ethanol production is provided by raw material nowadays (Rasmey et al., 2018).
Saccharomyces cerevisiae strain is the most widely used bio-ethanol producer organism. To enhance bioethanol production by yeasts with promising cost, different immobilized carriers are used (Laopaiboon et al., 2009). Production of bio-ethanol by immobilization has many technical and economic advantages compared to free cell system (Verbelen et al., 2006). The most widely used immobilization methods are based on cell entrapment in natural or polymers gels (Pan et al., 2010). The main drawbacks that control the use of polymer beads as cells carrier is problem of gel degradation, low physical strength, considerable substrate and product mass transfer limitation and gel particles disruption due to intensive CO2 evolution (Phisalophong et al, 2007; El-Gazzar et al., 2020). Other alternatives widely used method are based on passive adhesion of cells onto the surfaces of insoluble carrier such as glass beads, stainless steel wire spheres, sorghum bagasse (Yu et al., 2010; Razmovski ant Pejin, 1996; Tsakiris et al., 2004), sugar cane bagasse (Santos et al., 2008). However, in order to use this technology in bio-ethanol production, the immobilization carrier must be very cheap and cell immobilization should be achieved with minimal additional cost (Ogbonna et al., 2001). It was mentioned that immobilization of the yeast cells can avoid inhibition caused by high concentration of substrate and product, and also enhance bio-ethanol yield and productivity (Nikolić et al., 2009).
The objective of the current study was to maximize bio-ethanol production by optimizing the various factors governing its bio-synthesis while using suitable strains and raw materials.
MATERIALS AND METHODS
Microorganisms, feedstock and materials
About 6 yeasts isolates and one bacterial strain listed in (Table 1) were used for bio-ethanol production. The yeasts strains, bacterial strain were subcultured onto Sabouraud’s agar; nitrate agar (Oxoid) respectively (Enan et al., 2013, 2020; Osman et al., 2021a).
Two types of molasses (Table 2) were used as carbon source for bio-ethanol production by the given microbial strains. Egyptian sugar-cane molasses was provided by El Hawamdia factory (no. 1) and Cairo Factory (no. 2) for integrated sugar industry, Giza Governorate (20 Km West Cairo). While Egyptian sugar beet molasses was provided by El-Hamool sugar Factory, Kafr El-sheikh Governorate (120 Km, North Cairo).
Five different immobilizing carriers were used such as sodium alginate, agar–agar, carboxymethyl cellulose, sodium salt (Na–CMC) All of them obtained from HI-Media Co., Germany and artificial sponges, of loofa and fibers that were collected from mature dried fruit of Luffa cylindrica (Zagazig, Sharkia Governorate, Egypt).
Table 1: Experimental microbial strains used in this study.
Strains | Source | |
Saccharomyces cerevisiae | F514 |
National Research Centre |
Saccharomyces cerevisiae | ATCC4095 |
American Type culture collection, Ain Shams University, Egypt |
Candida kefyr | EMCC77 | |
Candida tropicalis |
EMCC2 |
Culture collection, Ain Shams University, Egypt |
Kluyveromyces marxianus |
NRRL1109 | |
Pichia stipites |
DSM3651 | |
Zymomonas mobilis |
ATCC31823 |
Table 2: Composition of sugarcane and beet molasses used in this study
.
Molasse s type |
Total protei n % |
Total sug ar (mg. ml-1) |
Organic carbo n % |
Tot al P % |
Ashes % |
Mineral substances mg .kg-1 |
|||||||
P |
C |
||||||||||||
C | O | b | Mn | Cr | Cu | Ni | d | Fe | |||||
Sugarcane molasses 1 | 6.01 | 3.57 | 47.56 | 0.12 | 12.74 | Nil | Nil | 13.13 | 0.76 | 8.8 | 1.91 | Nil | 345.3 |
Sugarcane molasses 2 | 11.44 | 4.46 | 42.98 | 0.012 | - | Nil | Nil | 15.86 | 3.38 | 7.93 | 7.52 | Nil | 366.46 |
Molasses pretreatment
About 1 liter of molasses was added to 0.5 L distilled water and then 0.001% concentrated sulfuric acid were added to remove the sludge and colloids, and pH value was adjusted to 4.0 using 0.1 N HCL by Automatic pH –meter (New Brunswek Science-Co.) (Enan et al., 2013). Later on, this mixture was heated in water bath to boiling and allowed to cool, then stand in refrigerator overnight and centrifuged at 3000 rpm for 15 min and the supernatant was used for further experiments (Sadik and Halema, 2014).
Sugar and bio-ethanol estimation
Dinitrosalycylic acid (DNS) method was used for estimation reducing sugar (Miller, 1959). Bio-ethanol production in the fermentable broth was measured by method (Nasir et al., 2017).
Immobilization of yeast strains
Sodium alginate, agar–agar, carboxymethyl cellulose sodium salt, artificial fiber sponges, and loofa sponges were used for immobilization of both S.cerevisiae and C. kefyr Ismaiel et al. (2015).
Fermentation process
For preparation of fermentation medium, about 250 mL, pretreated molasses; 3 g yeast extract; 0.1 g urea in 750 mL distilled water (Enan et al., 2013). About 50 ml of fermentation medium was controlled to pH 6 with 1M NaOH and sterilized at 121°C for 15 min. Then, inoculated with 5 ml of growing microbial culture containing 108.mL-1 CFU at 25°C and (150 rpm) for 3 days (Ismail et al., 2015).
Statistical analysis
Analysis of variance (ANOVA) was carried out using CoStat version 6.400 (CoHort software, Monterey, CA, 93940, USA). Mean values among treatments were compared by the Duncan test at 5% level (p. value < 0.05) of significance and presented as the mean values ± standard deviation (SD).
RESULTS AND DISCUSSION
Screening of bio-ethanol production by yeast and bacterial strains
Six yeast strains and one bacterial strain were surveyed for their ability to produce bio-ethanol from sugarcane and sugar beet molasses. The results given in Table 3 showed that all the tested yeast strains gave lower bio-ethanol production from beet molasses compared to that obtained from sugarcane molasses except for K. marxianus. On the other hand, these yeast strains were significantly (P. value 0.01) able to produce considerable amounts of bio-ethanol from sugarcane molasses. Out of the 7 yeast strains tested, 5 strains were able to produce more than or equal 5% bio-ethanol during the fermentation of sugarcane molasses. However, 2 strains only produced more than or equal 5% bio-ethanol during the fermentation of sugar beet molasses; the bio-ethanol amounts ranged between 50.6 and 62.4 g. L-1. It is worth to mention that the highest bio-ethanol producer strain on sugarcane and sugar beet molasses was S. cerevisiae followed by C. kefyr. These results conform to the findings of Singth et al. (2013). Al-Dulaimi et al. (2018) studied the ability of some yeasts to produce ethanol using dates, apricot and grapes, they found that alcoholic production obtained by S. cerevisiae was significantly higher than that obtained by C. kefyr. This is might be due to that S. cerevisiae is known to tolerate the growth in media rich with molasses and this could increase its production capability of bio-ethanol. However, C. kefyr is more preferable in kefyr fermentation (Al-Mohammadi et al., 2020).
Effect of initial pH on bio-ethanol yield
Initial pH value of fermentation broth had an influence on bio-ethanol production by yeast strains using two types of molasses as carbon source. Optimal pH value is necessary for the activity of plasma membrane-based proteins, including enzymes and transport proteins (Enan et al., 2013).
Table 3: Reducing sugar and bio-ethanol yield using two molasses as substrate.
Strains |
Sugar cane molasses |
Sugar beet molasses |
|||
Reducing sugar |
Bio-ethanol yield (g/L) |
Reducing sugar |
Bio-ethanol yield (g/L) |
||
S. Cerevisiae F514 |
40.8 ± 2.2b |
94.9 ± 0.8a |
8.74 ± 1.9c |
62.4 ±1.5a |
|
S. cerevisiae ATTCC4098 |
38.0 ± 0.4b |
52.4 ± 1.5c |
11.5 ± 0.5ab |
50.6 ± 0.7c |
|
C. kefyr |
47.8 ± 2.3a |
55.7 ± 0.7b |
13.7 ± 2.3a |
56.1 ± 0.4b |
|
C. tropicalis |
34.6 ± 3.2c |
54.6 ± 1.3b |
9.4 ± 0.6 bc |
29.4 ± 2.6 f |
|
K. marxianus |
37.7 ± 1.8bc |
9.87 ± 1.1e |
12.7 ± 2.4a |
34.7 ± 3.3e |
|
P. stipites |
34.9 ± 0.3c |
51.2 ± 0.2c |
4.58 ± 0.5d |
4.67 ± 0.3g |
|
Zymomon as mobilis |
31.6±2.5d | 45.8±0.96d | 12.2±0.21a | 44.2±3.5d |
Means ± standard deviation within a column followed by the same letter are not significantly different at P= 0.05 when compared by Duncan test.
Fermentation using the strain F514, was carried out at pH 4, 4.5, 5, 5.5 and 6.0 at 30°C, to determine the effect of pH on bio-ethanol production. Data given Figure 1 showed that bio-ethanol yield by S. cerevisiae from sugarcane molasses was higher at pH 4.5 (176.9 g L-1) than PH 5 (143 g L-1) and 6 (95.5 g L- 1). On the other hand, bio-ethanol yield by C. kefyr from the sugarcane molasses was higher at pH 5.5 (119.1 g L-1). From the data given in Figure 1 pH 4.5 and 5.5 were optimal for bio-ethanol production by S. cerevisiae and C. kefyr from sugarcane molasses respectively. However, pH 4 was the optimal pH value for bio-ethanol production by S. cerevisiae and C. kefyr from sugar beet molasses respectively. This result also in contradiction with that given in the study of El-Sayed et al. (2020) who reported that optimal pH for bio-ethanol production was around 4.5. The optimum pH value that induces the production of certain metabolite depends on the producer strain itself; different strains within certain one species or different species within one Genus could show different growth conditions (Enan et al., 1996; El-Sayed et al., 2020).

Figure 1: Effect of initial pH values on bio-ethanol production (g.L-1) by S. cerevisiae and C. kefyr using two kinds of molasses. (A) Sugarcane molasses (B) Sugar beet molasses Number followed by the same letter are not significantly different at P= 0.05 when compared by Duncan test.
Effect of different temperature incubation on bio-ethanol yield
The incubation temperatures showed marked effect on bio-ethanol production by the two strains using molasses as carbon source. To determine the effect of incubation temperature on bio-ethanol production of yeast strains, fermentation experiments were carried out at 25, 28, 30, 32 and 35°C using reducing sugar of sugarcane and sugar beet molasses. Bio- ethanol production gradually increased during fermentation temperature of 25 to 30°C and then sharply decreased with higher fermentation temperatures (Figure 2). At 32°C, S. cerevisiae and C. kefyr produced the highest yield of bio-ethanol using sugarcane molasses with superiority of S. cerevisiae in bio-ethanol production.
Moreover, growing of yeasts on sugar beet molasses showed maximum bio-ethanol production at 30 and 32 °C for S. cerevisiae and C. kefyr respectively. The yield of bio-ethanol at various temperatures indicated that the fermentation temperature 30°C was the optimum incubation temperature for production of bio-ethanol by two yeast strains on medium containing sugarcane molasses as carbon source. While, it was 30 and 30 and 32 °C for S. cerevisiae and C. kefyr, respectively. Bio-ethanol production by S.cerevisiae increased with the increase in temperature reaching the maximum value at 30°C (Figure 2). Further increase in temperature reduced the percentage of bio-ethanol production. These results contradict with the study of Yah et al. (2010) who found that the optimal temperature of bio-ethanol production was 25°C as S. cerevisiae IFST -072011 can produce bio-ethanol at higher temperature, it will be more suitable for industrial production of bio-ethanol. Hashem et al. (2021), studied the optimization of fermentation conditions for enhanced bioethanol yields by some yeasts. They found that the temperature of incubation has certain effect on bioethanol production; optimum temperature of incubation appeared to be in the range 30-35 oC. This indicated on the mesophilic nature of the yeasts.

Figure 2: Effect of incubation temperature on bio-ethanol production (g.L-1) by S. cerevisiae and C. kefyr using two kinds of molasses. (A) Sugarcane molasses (B) Sugar beet molasses Number followed by the same letter are not significantly different at P= 0.05 when compared by Duncan test at P= 0.05 when compared by Duncan test.
Effect of incubation periods on bio-ethanol production
Incubation periods showed significant distinctive on bio-ethanol production (P<0.05) by the investigated yeast strains using two types of molasses as carbon source. To assess the effect of incubation periods on bio-ethanol (Figure 3) production, fermentation processes were carried out for 1, 2, 3, 4 and 5 days using both sugarcane sugar beet molasses. The fermentation was done under optimal pH and temperature for two yeast strains that were obtained from the previous trials. Data in Table 4 observed that the bio- ethanol production was increased during incubation periods up to two days and then decreased again to reach the minimum levels at the fifth days of incubation. These results are real in fermentation process using two kinds of molasses by two yeast strains. The highest production of bio-ethanol was showed at the second day of fermentation. The production of bio-ethanol from sugarcane molasses reached 137.3 and 120.5 using S. cerevisiae and C. kefyr respectively. Moreover, the bio-ethanol production from sugarcane molasses was higher than its production from sugar beet molasses. (Table 5) Previous published results have indicated that the fermentation time greatly influence the biotechnol production by yeasts (Enan et al., 2018). It was approved that yeasts produce optimal values of bioethanol by fermentation of sugary substrates using actively growing yeast cells in the early to mid exponential phase of growth after 2 days of growth (El-Sayed et al., 2015) (Table 5).
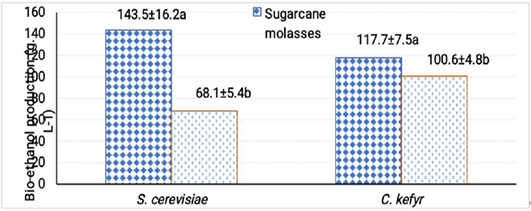
Figure 3: Effect of optimal conditions on bio-ethanol production by S. cerevisiae and C. kefyr using two kinds of molasses. Number followed by the same letter are not significantly different at P= 0.05 when compared by Duncan test.Section of intestine showing sloughing of villli and heterophillic infiltration)
Table 4: Effect of incubation period on bio-ethanol production by S. cerevisiae and C. kefyr using two kinds of molasses.
Incubation period (day) |
Sugarcane molasses |
Sugar beet molasses |
||
S. cerevisiae |
C. kefyr |
S. cerevisiae |
C. kefyr |
|
1 | 110±7.1b | 110.3±4.3a | 43.9±4.2d | 67.6±1.2c |
2 | 137.3±14.4a | 120.5±6.1a | 69.1±2.8a | 99.6.2±4.9a |
3 | 96.6±11.7b | 77.6±5.4b | 63.9±4.1ab | 76.8±2.6b |
4 | 70.7±3.6c | 64.2±8.6c | 61.9±1.2b | 62.2±0.7d |
5 |
54± 5.8c |
40.1±9.0d | 53.9±2.5c | 31.6±2.5e |
Means ± standard deviation within a column followed by the same letter are not significantly different at P= 0.05 when compared by Duncan test.
Effect of optimal conditions on bio-ethanol production
At the optimal fermentation temperature and initial pH, free cells of S. cerevisiae and C. kefyr were able to produce 143.5 and 68.1 g L-1 from sugarcane molasses, bio- ethanol after 48 h while gave 117.7 and 100.6 g L-1 from sugar beet molasses. Under all optimum condition S. cerevisiae gave bio-ethanol yield from sugarcane molasses higher than C. kefyr and vice versa in case of sugar beet molasses (El-sayed et al., 2015). Values of bioethanol produced were more or less similar to other published results as different factors could prevent the absolute similarly of bioethanaole produced such as nature of the yeast strains used, media, growth conditions and nutritional factors (Enan et al., 2018; Abdel Shafi et al., 2020).
Table 5: Effect of different carriers on bio-ethanol production by S. cerevisiae and C. kefyr using two kinds of molasses.
Carriers |
Sugarcane molasses |
Sugar beet molasses |
||
S. cerevisiae |
C. kefyr |
S. cerevisiae |
C. kefyr |
|
Loofa sponge |
164.5±4.7bc | 147.3±5.9c | 134.2±4.3c | 150.5±0.5c |
Artificial fiber sponge |
159.7±1.6c | 139.0±6.1c | 145.5±1.8b | 162.5±3.2b |
Agar agar |
161.2±5.2c | 150.6±5.1c | 142.6±2.3bc | 129.6±2.0d |
Na-CMC | 168.6±1.2b | 164.5±1.4b | 150.8±5.4b | 158±2.7b |
Sodium alginate |
200.8±3.4a | 198.1±10.3a | 178.9±9.0a | 196.1±5.4a |
Immobilization efficiency on bio-ethanol production
Immobilizations are of various advantages in industrial applications of yeasts. The most significant advantages of immobilized yeast cell systems is the ability to operate with high production yeild at different dilution rates that exceed the maximal specific growth rate; the increase of bio-ethanol yield and cellular stability and the decrease of process expenses due to the cell recovery and reutilization are which existed by this technique (El-Sayed et al., 2020). Immobilization of cells showed significantly effect on bio-ethanol production, as bio-ethanol production increased with immobilized cells. At optimum condition, maximal bio-ethanol production by S. cerevisiae was 143.5 and 117.7 g L-1 by free cells whereas 200.8 and 198.1 g L-1 bio-ethanol produced by immobilized cells using sodium alginate in the same condition at 48 hrs. regarding C. kefyr, the same trend of results was obtained. The data obtained herein have showed that the maximum production or bio-ethanol was obtained by immobilizing the cell on sodium alginate follow by Na-CMC. A study by Enan et al. (2020) supported this finding, where they concluded that immobilized S. cerevisiae produced higher concentration of bio-ethanol than free cells and within 24 h, as the immobilized S. cerevisiae strain could consume all the available sugar (Sing et al., 2013).
Conclusions and Recommendations
From the obtained results, it can be concluded that a successful fermentation process depends on sugar concentration of the medium and nutritional parameters. The maximal production of bio-ethanol by S. cerevisiae was obtained after 48 h of incubation with Sugarcane molasses adjusted at pH 4.5 and incubated at 32°C. At these conditions, 143.5 g L-1 bio-ethanol was produced by free cells against 200.8 g L-1 of bio-ethanol by immobilized cells. Concerning C. kefyr, the maximal production of bio-ethanol was obtained after 48 h of incubation with Sugarcane molasses, at pH 5.5 and 32°C by immobilized cells technique which was better for bio-ethanol production than free cells. These strains used (S. cerevisiae F514 and C. kefyr EMCC77 ) can be used for industrial production of bio-ethanol from different kinds of molasses.
Acknowledgements
I wish to express my sincere thanks, deepest gratitude and appreciation to the research team of a project “Bioconversion of lignocellulosic materials to produce biofuels” funded by the Scientific Research Account Fund at Benha University for offering every possible help during the preparation of this work. I thank Dr. A.A. Ismaiel, Faculty of Science, Zagazig University for his help in practical experiments.
Novelty Statement
The maximal production of bio-ethanol by S. cerevisiae.
Author’s Contribution
All authors have made asubstantial and intellectual contribution to the work, and confirmed it for publication.
Conflict of interest
The authors have declared no conflict of interest.
References