Advances in Animal and Veterinary Sciences
Research Article
Comparative Study between Wild and Commercial Egyptian Lactobacillus reuteri-Antagonizing Effects in Cryptosporidiosis Models
Azza Fahmy1, Gehan Abuelenain1*, Amr Abdou2, Mohamed Elhakeem3,4
1Department of Immunology and Drug Evaluation, TBRI, Giza, Egypt; *Affiliated with FCHS, IAT, P.O. Box 111534, UAE; 2Department of Microbiology and Immunology, NRC, Giza, Egypt; 3Water Resources and Environmental Engineering, ADU, Abu Dhabi, UAE; 4University of Tennessee, Knoxville, TN, USA.
Abstract | To investigate the prophylactic and therapeutic potentiality of Egyptian probiotic Lactobacillus reuteri in the cryptosporidiosis model (hereafter home-isolated probiotic). As per the health conditions of the experimental mice, they were deployed into two main batches: immunocompetent and immunocompromised. Each batch comprised three protocols as per the types of the treatment (home-isolated-, commercial probiotics, and Nitazoxanide). A dose of 108 colony-forming unit (cfu) Lactobacillus reuteri isolated from the domestic camels and the commercial strain were administered orally on daily basis for 10 days. Then, mice were infected with approximately 1 x 104 inoculums of Cryptosporidium cysts with continuous administration of probiotics till the end of the experiment (day 20). The regression decay models were utilized to predict the recovery rate of the experimental mice at days different from the tested ones using the data collected from the Nitazoxanide-different time-scheduled protocol. The response to the treatment by probiotics in the immunocompromised mice is less than that of the immunocompetent mice. Positive outcome results are in favor of the home-isolated probiotic treatment. On the contrary, Nitazoxanide is the treatment with the highest number of cysts recovered from the experimental mice. In a nutshell, the results demonstrate that the home-isolated probiotic is efficient in decolonizing the Cryptosporidium spp. cysts and ameliorating the pathogenicity of the disease. Besides, this research study is shedding the light on the importance of isolating and utilizing a country/region-based Lactobacillus reuteri probiotic for high-efficiency treatment of cryptosporidiosis in immunodeficient cases.
Keywords | Cryptosporidiosis; Nitazoxanide; Probiotic; Drug discovery
Received | February 19, 2021; Accepted | March 13, 2021; Published | April 15, 2021
*Correspondence | Gehan Labib Abuelenain, Department of Immunology and Drug Evaluation, TBRI, Giza, Egypt; Email: gehanlha@yahoo.com
Citation | Fahmy A, Abuelenain G, Abdou A, Elhakeem M (2021). Comparative study between wild and commercial egyptian lactobacillus reuteri-antagonizing effects in cryptosporidiosis models. Adv. Anim. Vet. Sci. 9(6): 802-810.
DOI | http://dx.doi.org/10.17582/journal.aavs/2021/9.6.802.810
ISSN (Online) | 2307-8316; ISSN (Print) | 2309-3331
Copyright © 2021 Abuelenain et al. This is an open access article distributed under the Creative Commons Attribution License, which permits unrestricted use, distribution, and reproduction in any medium, provided the original work is properly cited.
Introduction
Cryptosporidium spp. is the only genus of the subclass Cryptogregaria listed as a major foodborne protozoan parasite among Apicomplexa (Ryan et al., 2016). This intracellular parasite is transmitted by the ingestion of Cryptosporidium cysts through contaminated water and food ( Gerace et al., 2019). Its reproduction and gametes formation develop within the microvillus layer of intestinal epithelial cells in the host’s small intestine (Chen et al., 2005) causing watery diarrhea and malabsorption along with histopathological deterioration (Guitard et al., 2006). Persistent Cryptosporidium spp. infection is associated with villus atrophy, crypt hyperplasia, and variable increases in leucocytes in the lamina propria (Klein et al., 2008). The disease was reported with an extensive veterinary and medical impact worldwide (Salama et al., 2020) for being the main agent inducing moderate to severe diarrhea (Costa et al., 2020) in both immunocompetent and immunodeficient hosts (Chique et al., 2020), and due to causing malnutrition of the children of ages under 5-years old (Checkley et al., 2015). This opportunistic parasite can be life-threatening for patients with acquired immunodeficiency syndrome (Gerace et al., 2019), and hence, there is a need to develop or repurpose a drug to overcome the limitation of action of Nitazoxanide, which is not effective in for the treatment of cryptosporidium-induced diarrhea (Chen et al., 2002) So far, up to the authors’ best knowledge, there is no effective drug or vaccine that can eliminate cryptosporidiosis, despite the current promising results with the candidate therapy AN3661, which is still under investigation (Swale et al., 2019).
The approach of introducing probiotics to multiple gut-intestinal disorders has been well-perceived globally by scientists for the promising results recorded in some animal models and human research (Varankovich et al., 2015).
The current research study aimed at isolating and utilizing an Egyptian probiotic strain of L. reuteri (hereafter home-isolated probiotic) in experimental cryptosporidiosis. A commercial probiotic of the same strain, Nitazoxanide drug, and infected controls were the references used to evaluate the efficacy of this home-isolated strain.
Materials and methods
Ethical approval
This study was approved by the Ethics Committee of the Institutional Animal Ethics Committee and local laws and regulations of the National Research Centre, Egypt. Dealing with the experimental animals was carried out per the international valid guidelines and they were kept up under appropriate conditions at the Schistosome Biological Supply Program (SBSP) animal house of TBRI.
Mice, parasite, and infection
Parasite-free, 6-week-old male Swiss Albino mice (n=210) weighing 20-24 gm were used for this research study after their stool was examined for 3 consecutive days by the modified Ziehl–Neelsen stain technique (Tahvildar-Biderouni and Salehi, 2014). Cryptosporidium oocysts were obtained from the ileal mucous membrane and cecal content of naturally infected calves at slaughterhouses as described by Anderson (1985). Samples were preserved in an equal volume of 2.5% potassium dichromate (K2Cr2O7) as per the protocol of (Dubey, 2018). The infective inoculum was prepared, and cysts were counted as described by Ernst et al. (1982). The Cryptosporidium cysts were maintained by a regular passage in suckling rats (Capet et al., 1999), and purified through a sucrose gradient, and 1.25% sodium hypochlorite treatment just before inoculation. All overnight-thirsty experimental mice were infected orally and individually with approximately 1 x 104 inoculums of Cryptosporidium cysts, in 0.2 ml of sterilized phosphate buffer solution (PBS), after the priming phase.
Regimens
The experimental animals were deployed into two batches run in parallel, immunocompetent and immunocompromised, each with 105 mice. The home-isolated probiotic L. reuteri was courteously isolated, characterized, prepared as described in (Abdou et al., 2019) and provided by Dr. Amro Abdu, Department of Microbiology and Immunology, NRC, Giza, Egypt.
Probiotics administration in immunocompetent batch. The study was performed over 20 days. Post-priming phase, on day 11, all groups were infected by the prepared inoculums of Cryptosporidium cysts in 0.2 ml of sterilized PBS. Over the experimental 20-day duration, three groups of mice, 25 each, were assigned as infected controls administered with 0.2 ml PBS only (group I), commercial probiotic-treated mice administered with l08 colony-forming unit (cfu) in 0.2 ml PBS (group II), and home-isolated probiotic-treated mice administered with 108cfu in 0.2 ml PBS (group III). Fecal pellets of all mice were examined microscopically for any parasitic infection on the last day of the prime phase. Every other day post-infection (1, 3, 5, 7, and 9), five animals from each group were sacrificed by ether inhalation. Fecal samples were collected for Cryptosporidium enumeration. A 1-2 cm length of the proximal stomach, distal ileum, were removed from each mouse and fixed in 10% phosphate-buffered formalin (pH 7.4) for histopathological examination.
Probiotics administration in the immunocompromised experimental group. The previous 20-day treatment protocol was applied to the other 75 mice of this batch by considering day 15 post-Dexamethasone (DM) suppression as the zero-day. Dexamethasone (0.25 mg/g/day) was administered orally (Rehg et al., 1988). DM administration was maintained during the whole experimentation as previously described by Certad et al. (2007).
Nitazoxanide administration in immunocompetent and immunocompromised batches. Analogous to the previous protocol, sixty mice were deployed into two batches, 30 mice each. Fifteen immunocompetent mice were orally administered with 100mg/kg/day Nitazoxanide 3-days-post-infection for a week. The other 15-infected healthy mice served as controls. On the other hand, the immune system of the other 30 mice was crushed by Dexamethasone (0.25 mg/g/day) as previously described. On the 15th-day post-immune suppression, mice were infected with the prepared inoculums of cryptosporidium cysts and three days later, they were treated with Nitazoxanide (100mg/kg/day) for a week. The other 15-infected immunodeficient mice served as this batch’s controls. Five mice from each group were sacrificed on days 2, 5, and 8 post-treatment using ether inhalation.
Assessment instruments
Light Microscopic examination (LM) of Cryptosporidium cysts. A fecal smear was prepared and stained by Ziehl–Neelsen for the microscopy examination (Henriksen and Pohlenz, 1981). The total number of cysts/g was calculated as per a previous method (O’Handley et al., 1999).
Decay model. A decay model was developed for each experimental data set to indicate the recovery rate if any. The correlation between the cysts counts and the days after each probiotic treatment protocol was plotted using the Nitazoxanide protocol as a reference.
Histopathological examination. Paraffin sections were prepared as previously described (Cardiff et al., 2014). Sections of ilea were stained with hematoxylin and eosin (H &E) for evaluation.
Statistical analysis
Two-way ANOVA analysis was performed between the number of days after infection and the treatment protocol applied to all immunocompetent and immunocompromised mice.
Results
Microscopic counts of Fecal Cryptosporidium Spp. Cysts
The cysts were counted in all experimental mice at different intervals (1, 3, 5, 7, and 9 post-infection). The lower the number of cysts, the higher the likelihood that the mice are responding to the treatment. For the immunocompetent batch, the number of days after the infection had a statistically significant effect (p = 7.95E-17 compared to 0.01) on the recovery of the treated mice. Whilst the number of cysts dropped down by keeping the mice for a longer period under certain treatment protocols, a surge in the number of cysts was detected in the infected mice when kept over the same period with no treatment. As shown in Figure 1, the responses of experimental mice to the administration of the two probiotics were different (p = 2.4E-37 compared to 0.01). The home-isolated probiotic inoculation induced faster recovery (lower count of cysts) of mice compared to the one experienced with the commercial probiotic. Besides, the number of cysts recovered from the probiotics treated mice reached the peak on day 3, and gradually declined to reach the minimum on day 9, whereas their counterparts of the infected untreated mice

Figure 1: Count of Cryptosporidium cysts every other day post-infection of the groups of immunocompetent mice treated with a commercial- and home-isolated probiotics compared to the infected untreated controls. The thin vertical bars at each point refer to the standard errors of the tested samples.
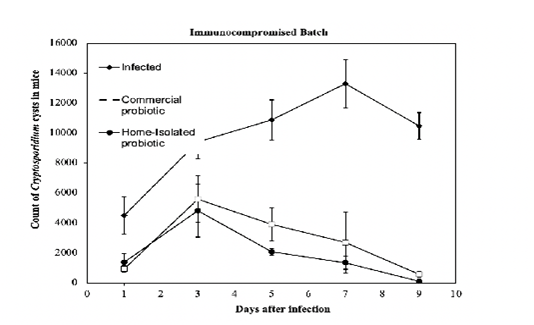
Figure 2: Count of Cryptosporidium cysts every other day post-infection of the groups of immunocompromised mice treated with a commercial- and home-isolated probiotics compared to the infected untreated controls. The thin vertical bars at each point refer to the standard errors of the tested samples.

Figure 3: Average count of Cryptosporidium cysts over the 10-day treatment phase for infected, immunocompromised, and immunocompetent mice. The thin vertical bars at each point refer to the standard errors of the tested samples.
reached the peak on day 7 and slightly declined on day 9. Although the values were slightly different, comparable results were observed for the examined immunocompromised mice (Figure 2). The thin vertical bars at each point in the graphs refer to the standard errors of the tested samples. It can be observed that immunocompromised mice (Fig. 2), in general, had a higher count of cysts compared to the immunocompetent mice (Fig. 1). This indicates that the response to the treatment by probiotics in the immunocompromised mice is less than that of the immunocompetent mice. On the other hand, the infected-untreated immunocompromised mice had a higher count of cysts compared to that one recorded in infected-untreated immunocompetent mice. The aforementioned observations are verified by the results shown in (Fig. 3), which depicts a comparison between the immunocompetent and immunocompromised mice under different regimens. The immunocompetent mice had lower average cysts count over the 10-day treatment phase compared to the immunocompromised mice. The thin vertical lines on the histogram columns refer to the standard errors of the tested samples.
Decay Models Results
In decay (regression) models, the declined data points respective to the number of days were considered rather than the days with the peak of cysts counts. These data points were considered within the range of 9 days starting on day 3 post-infection. The regression models were expressed for the commercial and isolated treatment protocols, respectively, as follows:
|
(1a) |
|
(1b) |
|
(2a) |
|
(2b) |
where t is the number of days after treatment, CC1 and CC2 are the counts of cysts of the immunocompetent and immunocompromised mice administered with the commercial probiotic mice, respectively, and CI1 and CI2 are the counts of cysts of the immunocompetent and immunocompromised mice treated with the home-isolated probiotics, respectively. Figures 4 and 5 show the experimental data fitted to the proposed decay regression models along with the coefficient of determination R2 for various models. The R2 value was about 0.98 for the various models. The high R2 value for all the proposed decay models indicates that there is a strong correspondence between the count of cysts of the mice and the number of days after treatment. The high R2 values prove also the reliability of the proposed models and that these decay models can provide satisfactory predictions for the count of cysts of the mice concerning the number of days after treatment for the examined protocols.
The regression decay models were utilized to predict the recovery rate of the experimental mice at days different from the tested ones based on the data collected from the Nitazoxanide-different time-scheduled protocol. In our research study, the regression models are valid for t ≥ 3, therefore for t = 2 simple average values were obtained from days 1 and 3 for the commercial- and home-isolated probiotics’ protocols. Figure 6 shows the three different treatments manifestation in the immunocompetent mice. Positive outcome results are in favor of the home-isolated probiotic treatment. On the contrary, Nitazoxanide is the treatment with the highest number of cysts recovered from

Figure 4: Fit of the regression decay models to the experimental data of the commercial probiotic. R2 value is the coefficient of determination.

Figure 5: Fit of the regression decay models to the experimental data of the isolated probiotic. R2 value is the coefficient of determination.

Figure 6: Treatment protocols for the immunocompetent mice compared to Nitazoxanide. The counts of Cryptosporidium spp. cysts on the days 2 and 8 post infection were calculated for the mice treated with the two probiotics using the decay model.
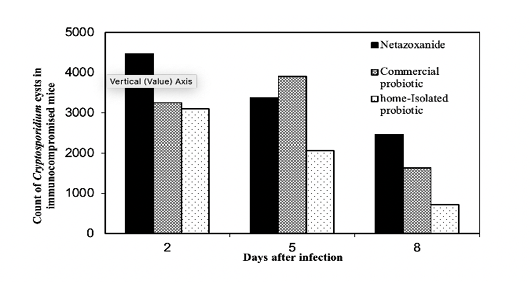
Figure 7: Treatment protocols for the immunocompromised mice compared to Nitazoxanide The counts of Cryptosporidium spp. cysts on the days 2 and 8 post infection were calculated for the mice treated with the two probiotics using the decay model.
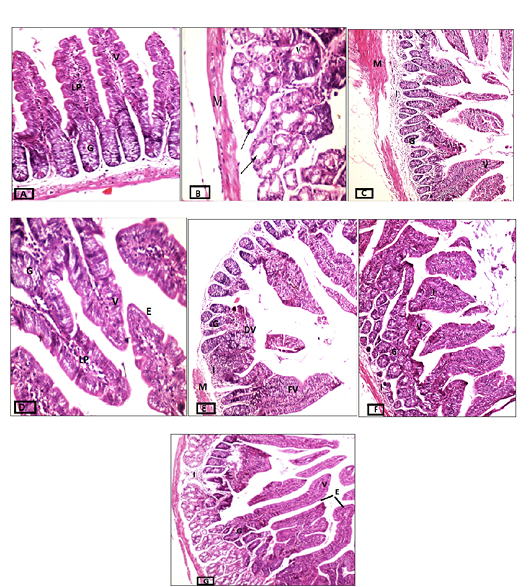
Figure 8: Cross-sections of intestinal tissues stained with hematoxylin and eosin. A) Control group, magnification 20x. B) Infected immunocompetent mice, magnification 40x. C) Immunocompetent mice infected and treated with the commercial probiotic, magnification 10x, D) Immunocompetent mice infected and treated with home-isolated probiotics, magnification 40x. E) Infected immunocompromised mice magnification 10x. F) Immunocompromised mice infected and treated with commercial probiotic magnification 20x. G) Immunocompromised mice infected and treated with home-isolated probiotic magnification 10x. Villi (V), Lamina Propria (LP), Goblet Cells (G), Degenerated Villi (DV), Fused Villi (FV), and Cell Infiltrate (I).
the experimental mice. Similar trends can be observed in the immunocompromised mice, however, the number of cysts of mice, in general, is higher for the immunocompromised mice as could be seen in Figure 7.
Histopathological changes
The intestinal tissues of various probiotic-treated mice were microscopically examined for estimating the effect of those treatments during the different health conditions (competent vs deficient). Tissues of normal mice had a discernible, regular continuous brush border on the columnar epithelium with intact villi (V), Lamina propria (LP), and goblet cells (G), which are noted at intervals between the surface columnar cells (fig. 8A). Challenging the immunocompetent mice with the cryptosporidiosis resulted in some pathological effects noticed in atrophied, short, fused (V) and degenerative changes to secretory glands (arrow), and muscularis (M) as shown in (fig. 8B), whilst on the other hand, treating those infected immunocompetent mice with commercial probiotic considerably ameliorated their intestinal tissue (fig. 8C), and this improvement was best attained by the home-isolated probiotic’s treatment (fig. 8D). In the latter group, most of the villi were integral with their tall columnar epithelium and mild inflammatory cells in their core. On the other hand, the intestinal tissues of infected immunocompromised mice experienced degenerated and fused villi (DV and FV, respectively) with pathological alterations in focal areas, severe vacuolar degeneration in mucosal epithelial cells, and mild inflammatory cell infiltrate in the submucosal layer (I). Also, goblet cells were remarkably diminished compared to those of the normal intestinal tissues (fig. 8E). The intestinal tissues of the infected immunodeficient mice treated with commercial probiotics showed a marked reduction in goblet cells and mild to moderate pathological alterations (Fig. 8F) reflected on the focal area of considerable degenerative glandular changes and mild inflammatory cell infiltrate in the submucosal layer (I). Many villi (V) also showed normal tall columnar epithelium with moderate inflammation in its core. Similarly, the infected immunodeficient mice treated with isolated probiotic maintained mild pathological changes in the tissue structure. Although intact glands with many villi (V) were detected, some villi displayed fusion with proliferated tall columnar epithelium (E) at the tip of the villi, together with the reduction of goblet cells.
Discussion
In this study, the experimental mice received either the Egyptian L reuteri strain or the same commercial strain developed without observed complications or signs of toxicity, which is supported by other studies (Walter et al., 2011; Hou et al., 2014; Yang et al., 2015; Liu et al., 2017).
Since the stringent challenge of applying Nitazoxanide against cryptosporidiosis is recorded with the immunodeficient cases, we designed our protocol to include immunosuppressed animals alongside the immunocompetent ones, when Dexamethasone was the immunosuppressive drug of our choice based on several previous studies (Diehl et al., 2017; Giles et al., 2018; Strehl et al., 2019). The Swiss Albino adult mice were used due to their availability and comparatively safe to handle. Being affordable, the big number of mice enabled us to ensure the Cryptosporidium infection and run the experiment in a long term to record the cysts at variable times. Oral inoculation of Cryptosporidium spp. cysts to the experimental mice was a simulation of cysts ingestion in natural infection which is supported by Bouzid et al. (2013) and the number of cysts used to develop the disease in our model was 1 x 104/ml, which is the acceptable range, in general, to create an infection in mice (Benamrouz et al., 2012).
We observed the cysts shedding of the infected (un-/treated) mice in all groups started at day 1 post-infection (PI), which is supported to a certain extent by (Nossair et al., 2018) and different from (Zambriski et al., 2013). These controversial findings may be due to the differences in animal species and microbiota. In general, the rate of cysts shedding was higher in the immunocompromised mice than their counterparts in the immunocompetent mice, which indicates a higher rate of autoinfection cycle of Cryptosporidium spp. in the intestinal epithelial cell membranes due to the suppression of anti-parasite CD4+ cell immune responses (Gebrewahid et al., 2019) by dexamethasone (Giles et al., 2018). The maximum numbers of fecal cysts excreted by the treated and untreated mice were attained on days 3 and 7 PI, respectively. There was a pattern of an indirect relationship between the number of fecal cysts shedding and the term of infection; the longer the time post-infection, the less the number of fecal cysts excreted by probiotics-treated mice in the immunocompetent and immunocompromised batches. The cysts’ shedding declined and almost ceased at day 10 PI, accordingly there was no need to extend the experimental plan for a longer time post-infection. The peak of fecal cysts shedding correlated with the time after infection varies from one study to another as per the model, gender, cysts genotype, source, and dose inoculum, etc. (Nossair et al., 2018), therefore the comparison is too difficult. In our study, we used the cysts of Cryptosporidium spp., which were isolated from Egyptian animals and prepared in our lab. This might raise the question that the period of cysts shedding is a strain-dependent, then, a future comparative study has to be performed. On the other hand, the remarkable progressive decline of fecal cysts shedding since day 3 in the probiotics-treated immunocompetent mice indicates an antagonizing effect of the L. reuteri on cryptosporidiosis (Al-alousi and Abdullah, 2018). Scientifically speaking, the probiotics might block the pathways to the epithelium cells infection by competing for the pathogen-receptor binding sites and /or changing the microenvironment of the pathogen by generating metabolites such as tryptophan and histamine-related enzymes like lactase and bile salt hydrolases that create an unpleasant experience to the parasite and restore gut flora (Oelschlaeger, 2010).
Interestingly, the recovery speed was accelerated in immunocompetent and immunocompromised mice by the home-isolated probiotic rather than by the commercial probiotic as indicated by the average number of fecal cysts shedding. This positive influence might be attributed to the compatibility of Egyptian Lactobacillus reuteri to the gut microbiome of the experimental mice which together with the unified and consistent environmental factors strengthen the action of this bacterium strain as anti-cryptosporidium (Terrapon and Henrissat, 2014).
In the current study, we treated the immunocompetent and immunosuppressed mice with Nitazoxanide, as a well-studied and approved drug reference, implementing a different schedule to use the data for predicting the number of fecal cysts shedding in the hidden days (2, 4, 6, and 8), and hence, to verify the correlation between the number of fecal cysts and infection duration. Surprisingly, the home-isolated probiotic diminished the average number of fecal cysts showing a superior effect over the commercial probiotic and the Nitazoxanide in both immunocompetent and immunocompromised batches. While Nitazoxinde acts directly on the parasitic-protozoa by destructing their cell membranes including those of the mitochondria causing inhibition of the pyruvate, ferredoxin/flavodoxin oxidoreductase (PFOR) cycle (Broekhuysen et al., 2000). The probiotics act naturally to enhance the immune system and indirectly compete with the pathogens resulting in their clearance from the gut (Mathipa et al., 2017). These different action modes may be in favor of using the probiotics either alone or combined with Nitazoxanide because the latter does not prevent the autoinfection, whilst the former does by preventing the cysts to inhabit the epithelium cells.
The histopathology of intestinal tissues showed ileum burdened with the cysts and severe microvillus damage, edematous and vacuolated epithelial cells in the infected untreated mice of both immunocompetent and immunosuppressed batches. These results are supported by others’ findings (Certad et al., 2007; Abdou et al., 2013). However, treating those infected mice with the two probiotics remodeled the morphology and cellular configuration of intestinal microvilli and epithelium cells. Moreover, the decolonization of Cryptosporidium cysts in the intestinal tissues of those probiotics-treated mice was remarkable. This may be, fortunately, due to the low pH microenvironment cherished by Cryptosporidium spp. and endured by L. reuteri probiotics (Krumbeck et al., 2016). Whilst the conditions of the upper small intestine were ideal for the propagation of the parasite, the biological tolerance of L. reuteri enabled it to colonize and efficiently compete Cryptosporidium cysts for adhering themselves to mucin and intestinal epithelia kicking the cysts out to the lumen.
Conclusion
This research paper with its findings demonstrates that the Egyptian L. reuteri strain may be effective for the prevention of Cryptosporidium spp. in immunocompromised and immunocompetent subjects. Further and future work will use the same protocol to understand the concomitant T and B cells immune responses including IFN-gamma and IL-10 as well as the IgA.
Acknowledgments
The pathological photographs and comments were mentored and audited by Dr. Sahar Darwish, Professor, and Head of Pathology Department in the National Organization for Drug Control and Research, Egypt. Deep gratitude and thanks to Dr. Darwish for her valuable assistance.
Conflict of interest
The authors have declared no conflict of interest.
Authors contribution
All authors contributed equally.
References