Advances in Animal and Veterinary Sciences
Research Article
Breast Milk MSCs Transplantation Attenuates Male Diabetic Infertility Via Immunomodulatory Mechanism in Rats
Tarek Khamis1, Abdelalim F. Abdelalim1, Somia Hassan Abdallah2, Ahmed A. Saeed1, Nagah M. Edress1, Ahmed Hamed Arisha3*
1Department of Pharmacology, Faculty of Veterinary Medicine, Zagazig University, 44519 Zagazig, Egypt; 2Department of Biochemistry, Faculty of Medicine, Zagazig University, 44519 Zagazig, Egypt; 3Department of Physiology, Faculty of Veterinary Medicine, Zagazig University, 44519 Zagazig, Egypt.
Abstract | Diabetes is considered one of the most progressive metabolic syndrome that affects up to 7% of the people worldwide with many complications. Although the exact molecular mechanisms involved are still controversial, diabetic infertility represents a common complication and, in this regard, recent research offers a promising candidate where mesenchymal stem cells have been applied in dealing diabetes and its complications. The present study investigated the ameliorative effect of breast milk mesenchymal stem cells (Br-MSCs) in diabetic testicular dysfunction rat model with emphasis on testicular immunomodulation and proliferating cells nuclear antigen (PCNA) expression. Forty-five adult male Sprague Dawley rats (8-12 weeks old) were divided into three groups such as control (n=15), diabetic (n=15) and diabetic-treated and, 14 days post induction of type 1 diabetes (n=15), were administered double intraperitoneal injection of Br-MSCs. Testicular gene expression interleukin 1 beta (IL1β), interleukin 6 (IL6), interleukin 8 (IL8), proliferating cell nuclear antigen (PCNA), transforming growth factor beta (TGFβ1) and interleukin 10 (IL10) were determined. Br-MSCs administration elicited a significant improvement in sperm count (P < 0.01), motility (P < 0.01) and abnormality (P < 0.01), a significant reduction (P < 0.001) in pro-inflammatory markers IL1β, IL6, IL8, and TGFβ1, a significant increase (P < 0.001) in anti-inflammatory testicular marker IL10, and the cell proliferation marker PCNA. The study conclude that Br-MSCs could ameliorate diabetic infertility via immunomodulatory effect and create a local anti-inflammatory microenvironment that reduced further testicular dysfunction.
Keywords | Br-MSCs, Type1 diabetes, Interleukins, PCNA, TGFβ1
Received | September 19, 2019; Accepted | October 26, 2019; Published | December 12, 2019
*Correspondence | Ahmed Hamed Arisha P.h. D. Department of Physiology, Faculty of Veterinary Medicine, Zagazig University, 44519 Zagazig, Egypt; Email: vetahmedhamed@zu.edu.eg; tarekkhamis13@yahoo.com
Citation | Khamis T, Abdelalim AF, Abdallah SH, Saeed AA, Edress NM, Arisha AH (2019). Breast milk MSCs transplantation attenuates male diabetic infertility via immunomodulatory mechanism in rats. Adv. Anim. Vet. Sci. 7(s2): 145-153.
DOI | http://dx.doi.org/10.17582/journal.aavs/2019/7.s2.145.153
ISSN (Online) | 2307-8316; ISSN (Print) | 2309-3331
Copyright © 2019 Khamis et al. This is an open access article distributed under the Creative Commons Attribution License, which permits unrestricted use, distribution, and reproduction in any medium, provided the original work is properly cited.
INTRODUCTION
Diabetes represents one of the most prevalent complication of metabolic syndrome that affect around 7% of people worldwide. It has several health impacts such as diabetic infertility (Melendez-Ramirez et al., 2010). Several mechanisms were incremented in induction of testicular damage; one of them is local testicular inflammatory condition potentiated by hyperglycemia (Chandrashekar and Muralidhara, 2009). On the other hand, diabetes-induced-testicular dysfunction via generating inflammatory environment is common complication involving an upregulation in the expression of pro-inflammatory cytokines such as TNF, IL1B, IL6 and IL8 (Bornstein et al., 2004; Leisegang and Henkel, 2018). Many authors reported an elevated level of pro-inflammatory cytokines including TNFα (Bornstein et al., 2004), IL1β (Svechnikov et al., 2001) and IL6 (Hales et al., 1999) in association with testicular damage. Also, in an in vitro study, Leisgang and Henkel in (2018) reported that the pro-inflammatory cytokine induced cellular damage in a dose dependent manner (Leisegang and Henkel, 2018). It has also been reported that chronic inflammation downregulated testicular steroidogenesis via modulation of both sertoli and leydig cell function (Bornstein et al., 2004). In addition, there has been accumulating evidence suggesting that the pro-inflammatory cytokines not only affect male fertility peripherally, but also it does centrally by modulating HPG (Tremellen et al., 2018).
Though complicated, mesenchymal stem cells transplantation is considered one of the best promising strategies for overcoming diabetes and its complications. The different types mesenchymal stem cells (MSCs) possess varying therapeutic potential depending on its source. Breast milk represents a non-invasive rich source for stem cells specially MSCs (Patki et al., 2010). Br-MSCs possess a higher therapeutic potential compared to other types of MSCs since it expresses many embryonic stem cells nuclear factor such as octamer binding transcription factor 4 (OCT 4), NANOG and sex determining region Y HMG/box 2 (SOX2) (Hassiotou et al., 2012). Several recent research has evidenced that MSCs therapy prevent and even revert diabetic testicular dysfunction (Karimaghai et al., 2018; Monsefi et al., 2013). This may be owing to MSCs secretion of many trophic factor that promotes local immunomodulation and induces anti-inflammatory via a paracrine effect in response to reduced diabetic testicular damage (Kim et al., 2015). The main objective of this study is to investigate the ameliorative effect of Br-MSCs in diabetic testicular dysfunction rat model with emphasis on testicular immunomodulation and proliferating cells nuclear antigen (PCNA) expression.
Materials and Methods
Collection of breast milk sample, preparation and culture of Br-MSCs
The milk samples were collected as aseptically as possible from breast feeding unit in the Department of Pediatrics, Zagazig University hospitals with a written consent from nursing mothers that were parturiated 2 to 5 days before (Patki et al., 2010). The preparation and culturing procedures were performed according to the protocol described previously (Patki et al., 2010). Briefly, breast milk was diluted 1:2 with high glucose DMEM (4.5 g/L glucose with L-glutamine, Lonza Bioproducts Walkersville, MD 21793-0127 USA) containing a mixture of penicillin-streptomycin-Amphotericin B as 10 IU/10 IU/25 mg (Lonza Bioproducts Walkersville, MD 21793-0127 USA) and centrifuged at 285g for 10 min. The cell pellet was washed twice with sterile phosphate-buffered saline (PBS) (Lonza Bioproducts Walkersville, MD 21793-0127 USA). Final cell pellet was seeded in a 25 cm2 tissue culture flasks containing DMEM medium, 10% fetal bovine serum (FBS) and penicillin-streptomycin-amphotericin B (Lonza Bioproducts Walkersville, MD 21793-0127 USA). The flasks were incubated at 37°C under 5% CO2 and 95% relative humidity in CO2 incubator (Heraeus, Hanau, Germany). The medium was changed every 48 hrs for 14 days as primary passage. At confluency of 80%, cells were trypsinized with 0.25% trypsin containing 0.02 % EDTA (Lonza Bioproducts) for 5 min at 37 oC and centrifuged at (2400 rpm for 20 min). Cells were counted with hemocytometer and their viability was conducted with trypan blue stain. Washed cell pellet was re-suspended in DMEM prior to transplant.
MSCs identification
Br-MSCs were identified according to the method previously described (Patki et al., 2010) based upon their adhesiveness to bottom of the culture flask and their fusiform, star or spindle shape under an inverted microscope. Also, MSCs were immunophenotyped with flow cytometry. The isolated cells were positive for MSCs surface marker such as CD105, CD90 whereas were negative to hematopoietic markers such as CD34, CD45. The cells from the 3rd passage was detached with 0.05% trypsin 0.02% EDTA in PBS. The cells were suspended in DMEM and fixed in chilled 70% ethanol. The cells were then incubated with mouse antihuman fluorescein isothiocyanate (FITC)/ phycoerythrin (PE) conjugated antibodies against CD45, CD34, CD90 and CD105 (Becton Dickinson, San Diego, CA, USA) at a dilution rate of (1:100) for 1 hr. The cells were identified with flow cytometer laser 488 nm (Becton Dickinson, New Jersey, NJ, and USA) with 10,000 gated cells. Data were analyzed using BD Cellquest Pro software (BD Bioscience Bedford, MA, USA) with forward and side scattered gate.
Experimental animals
Healthy forty-five adult male Sprague Dawley rats (8-12 weeks old, 250 to 300 gm) were purchased from laboratory animal house facility at the faculty of Veterinary Medicine, Zagazig University, Egypt. All experimental procedures were approved by the Institutional Animal Care and Use Committee (Approval no: ZU-IACUC/2/F/124/2019). Experimental animals were housed at 24 oC and were fed standard pelleted feed and water ad-libitum. Experimental animals were kept as such for 10 day for acclimatization before any experimental procedures.
Experimental designs
The experimental rats were randomly assigned to one of three groups; control (n=15), diabetic (n=15) and diabetic treated with Br-MSCs (n=15). Type 1 diabetes was induced with a single intra-peritoneal injection of STZ 65 mg/kg bodyweight as per description made previously (Wu and Huan, 2008).
Injection of Br-MSCs
The adherent MSCs were trypsinized, washed and resuspended in PBS (Lonza Bioproducts Walkersville, MD 21793-0127 USA). Two weeks after induction of type 1 diabetes with 14-days interval, cells were exposed with double intraperitoneal injections of 0.5 ml PBS containing 2×107 Br-MSCs. The same volume of PBS was injected into diabetic untreated and control groups.
Sample collection
At the end of the experiment (12 weeks post STZ injection), blood samples were collected via median eye can thus with/without anticoagulant for separation of plasma and serum. After that all experimental animal were sacrificed by decapitation followed by exsanguination testis were collected and divided into 2 parts; one for histopathological examination in 10% neutral buffered formalin solution and another one for total RNA extraction (30 mg) for gene expression analysis. Blood glucose was assayed by glucometer (URight blood glucose meter TD-4251). The serum insulin level was measured by commercially available specific rat insulin ELISA kit (RayBiotech) according to manufacturer instruction. The absorbance (at 450 nm) was measured using ELISA reader (DNM–9602; Beijing Perlong Medical Instrument Ltd., China).
Sperm quality analysis
At the end of the experiment, the epididymis was excised and macerated in a petri dish containing 10-ml Hank’s balanced salt solution at 37 oC. For sperm motility, one drop of the resulting solution was examined directly under light microscope for three grades (non-motile %, motile but not progressive % or motile and progressive %) at 400X. Sperm abnormality and live dead ratio were assayed using Eosin-Nigrosine stain. Sperm head and/or tail abnormalities were counted per 100 sperm. Total sperm count was assayed by diluting (1:10) the sperm suspension with 10% formaldehyde fixative (Sigma, USA) and analyzed through hemocytometer (Roshankhah et al., 2019).
Gene expression studies by real-time PCR (qPCR)
Total RNA was extracted from 30 mg of rat testis with Trizol reagent (Thermo Fisher Scientific; Waltham, MA, United States) followed by a two-step real-time PCR to evaluate gene expression as reported previously (Arisha et al., 2019; Khamis et al., 2020). Briefly, cDNA synthesis used by a Hi Sen Script™ RH (-) cDNA Synthesis Kit (iNtRON Biotechnology Co., South Korea) and rea-time PCR involved mixture 5X HOT FIRE Pol Eva Green qPCR Mix Plus (Solis BioDyne, Tartu, Estonia) and specific primers (Table 1). The reaction was carried out in a Veriti 96-well thermal cycler (Applied Biosystems, Foster City, CA) and cycling conditions consisted of initial denaturation at 95 °C for 12 min, followed by 40 cycles of denaturation at 95 °C for 30 sec annealing at 60 °C for 60 sec, and extension at 72 °C for 60 sec. The relative expression level of the target genes was normalized to that of the housekeeping GAPDH, and the relative fold changes in gene expression were calculated based on the 2−ΔΔCT comparative method (Livak and Schmittgen, 2001).
Histopathology of testes
Collected testes were fixed in 10% neutral buffered formalin solution. Following the proper histological procedures, the testicular tissue was embedded in paraffin wax according to previously described protocol (Alam et al., 2019; Arisha and Moustafa, 2019; Layton and Suvarna, 2013) and eventually sectioned (5 µm), stained with HandE and examined under light microscope (100X).
Data statistics
Statistical analysis was done with GraphPad Prism 8 software (San Diego, CA, United States). Data were expressed as mean±SEM. Comparison between group were done by one-way ANOVA followed by Tukey Post hoc tests. P < 0.05 was considered significant.
RESULTS
Br-MSCs identification
On 3rd day, the isolated cells showed typical MSCs characters such as rounded and elongated nuclei (Figure 1A). At 7th day of culture, the cells showed typical fibroblastic appearance (Figure 1B). The isolated cells showed typical MSCs phenotypical characters as cells appear to be positive for the mesenchymal stem cell surface markers CD90 (Figure 1E) and CD105 (Figure 1D) and negative for hematopoietic stem cells surface marker CD34 and CD45 (Figure 1F).
Effect of Br-MSCs administration on the glycemic index of type 1 induced diabetic rats
The result of the present investigation indicated that induction of type1 diabetes results in a significant increase the fasting blood glucose while a significant decrease in serum insulin compared to the control group (Figure 3). On the other hand, Br-MSCs administration elicited a significant reduction (P < 0.001) in the mean value of blood glucose level compared to diabetic group (Figure 3A). Also, there was a significant increase (P < 0.001) in the mean value of serum insulin level of Br-MSCs treated group compared to diabetic one (Figure 3B).
Effect of Br-MSCs administration on sperm picture of type 1 induced diabetic rats
The result of our investigation indicated significant (P < 0.001) decreases in sperm count and sperm motility in
Table 1: Primers Sequences used for real time PCR.
Gene |
Forward primer (5′–3′) |
Reverse primer (5′–3′) |
Accession No | Product size |
Gapdh | GGCACAGTCAAGG CTGAGAATG |
ATGGTGGTGAAG ACGCCAGTA |
NM_017008.4 | 143 |
IL1β |
CACCTCTCAAGCAG AGCACAGA |
ACGGGTTCCATG GTGAAGTC |
NM_031512.2 | 81 |
IL6 | ATATGTTCTCAGGG AGATCTTGGAA |
GTGCATCATCGCTG TTCATACA |
NM_012589.2 | 80 |
IL8 | CATTAATATTTAA CGATGTGGATGCGTTTCA |
GCCTACCATCTTTA AACTGCACAAT |
NM_030845.1 | 76 |
IL10 | GTAGAAGTGAT GCCCCAGGC |
AGAAATCGATGA CAGCGTCG |
NM_012854.2 | 116 |
PCNA | GAGCAACTTGGAAT CCCAGAACAGG |
CCAAGCTCCCCACT CGCAGAAAACT |
NM_022381.3 | 158 |
TGFβ1 |
CTGAACCAAGGAG ACGGAAT |
GGTTCATGTCA TGGATGGTG |
NM_021578.2 | 142 |
Table 2: Effect of Br-MSCs administration on sperm count, motility and abnormalities % in type 1 diabetic rats.
Control | Diabetic | Treated | |
Sperm count × 106 |
|||
Sperm count × 106 |
12.67 ± 2.52a |
5.13 ± 0.81b |
9.8 ± 0.92a |
Sperm abnormality (%) | |||
Head abnormality (%) |
5.33 ± 0.45b |
25.33 ± 2.08a |
6.67 ± 1.53b |
Tail abnormality (%) |
3.67 ± 0.38c |
20.00 ± 1.58a |
14.33 ± 1.38b |
Head and tail abnormality (%) |
1.670 ± 0.15c |
23.67 ± 1.53a |
9.00 ± 0.86b |
Sperm motility (%) | |||
Non motile (%) |
5.41 ± 0.43c |
62.75 ± 5.23a |
21.07 ± 1.25b |
Motile but not progressive (%) | 4.83 ± 0.35 | 4.55 ± 0.26 | 9.48 ± 0.85 |
Motile progressive (%) |
89.76 ± 1.25a |
32.70 ± 2.75c |
69.45 ± 5.78b |
AbcMeans ± SEM at the same row and bearing different superscripts are significantly different at p < 0.05.

Figure 1: (A.) Br-MSCs isolation 3rd day of culture showed rounded and elongated nucleus (B.) 7th day of culture cells showed typical fibroblastic appearance. Br-MSCs flow cytometrical identification (C.: F.) (C.) side scatter and forward scatter gated Br-MSCs cells, (D.) gated Br-MSCS cell population were positive for CD105, (E.) gated Br-MSCS cell population were positive for CD90 (F.) gated Br-MSCS cell population were negative for hematopoietic stem cell surface marker (CD34, CD45).
diabetic group compared to control group (Table 2). Also, diabetic group showed a significant (P<0.001) increase in sperm abnormality compared to control group (Table 2). However, Br-MSCs administration elicited a significant increase (P<0.001) in sperm count and sperm motility (Table 2). In addition, Br-MSCs treated group showed a significant (P<0.001) decrease in sperm abnormality compared to diabetic group (Table 2) (Figure 2A-I).
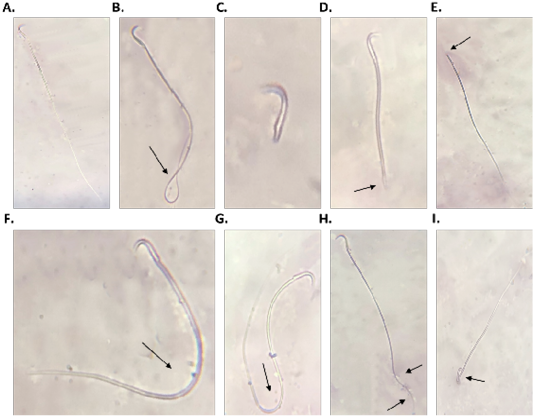
Figure 2: Sperm morphology (A.: I.), A. normal sperm, B. looped tail sperm, C. detached tail sperm, D. stunt sperm, E. detached head, F. and G. bent tail H. curved tail sperm, I. broken head.
Effect of Br-MSCs administration on the testicular expression of different cytokines and cell proliferation marker of type 1 diabetic rats
A significant upregulation in relative mRNA expression of local testicular inflammatory markers was observed for IL1β (P<0.001) (Figure 3C), IL6 (P<0.001) (Figure 3D), IL8 (P<0.001) (Figure 3E), and TGF1β (P<0.001) (Figure 3F) compared to control group. On the other hand, diabetic rat showed a significant downregulation in local testicular anti-inflammatory marker IL10 (P<0.01) (Figure 3H) and the cell proliferation marker PCNA (P<0.001) (Figure 3G) compared to control group. In contrast Br-MSCs intraperitoneal administration elicited a significant downregulation in relative mRNA expression of local testicular inflammatory markers IL1β (P<0.001) (Figure 3C), IL6 (P < 0.001) (Figure 3D), IL8 (P < 0.001) (Figure 3E), and TGF1β (P<0.001) (Figure 3F) compared to diabetic group. In addition, Br-MSCs treated rats showed a significant decrease in local testicular anti-inflammatory marker IL10 (P<0.001) (Figure 3H) as well as the cell proliferation marker PCNA (P<0.001) (Figure 3G) compared to diabetic group.
Effect of Br-MSCs administration on testicular histology of type 1 diabetic rats
The results of histopathological examination of H and E stained testicular section of diabetic rats showed severe interstitial edema with either complete or partially atrophied seminiferous tubules (Figure 4B). In contrast to Br-MSCs treated diabetic rats, examination of H and E stained testicular section revealed mild to moderate interstitial edema with functionally active seminiferous tubules and hypertrophied Leydig cells (Figure 4C).
DISCUSSION
Nowadays MSCs therapy represents one of the best strategies for controlling or even managing chronic non-curable diseases as it regenerates damaged tissue involving various mechanisms. However, its therapeutic potential depends mainly on the type and source of the stem cells. In our study, we selected Br-MSCs because they were easy to obtain with non-invasive technique unlike the other sources of adult MSCs such as bone marrow and adipose tissue derived MSCs. Furthermore, higher therapeutic potential has been shown for Br-MSCs compared to other MSCs candidates as it expresses many embryonic stem cells nuclear marker such as OCT4, Nano G and SOX2 (Hassiotou et al., 2012; Hassiotou and Hartmann, 2014; Ninkina et al., 2019; Patki et al., 2010). Collectively, it represents an ideal alternative for the other MSCs candidates that were used in the past years. In the current study, isolated Br-MSCs showed typical character of MSCs as it can adhere to the bottom of the culture flask, showing rounded to elongated nuclei at 3rd of culture and a typical fibroblastic appearance at 7th days of culture. Also, it showed the proper phenotype for MSCs with flow cytometrical analysis of the isolated cells; positive for the surface markers CD105 and CD 90 and negative for hematopoietic stem cells CD45 and CD34 (Patki et al., 2010).

Figure 3: Effect of Br-MSCs administration on blood glucose level, serum insulin level and testicular immune profile of type 1 induced diabetic rat (A-H). A. fasting blood glucose level, B. serum insulin level and RT-PCR analysis for relative mRNA expression of; C. IL1β, D. IL6, E. IL8, F. TGFβ1, G. PCNA and H. IL10 gene in testicular tissue. Means bearing different superscripts were significantly different at P < 0.05. Abbreviations; IL (interleukin), TGFβ1 (Transforming growth factor beta 1), PCNA (proliferating cell nuclear antigen) Gapdh (Glyceraldehyde 3-phosphate dehydrogenase).

Figure 4: Effect of Br-MSCs administration on testis morphometry of type 1 induced diabetic rats (A – C): A. Photomicrograph of testes showing normal testicular tissue with preserved seminiferous tubules (black arrow) and leydig cells (yellow arrow) HandE X 100, B. Photomicrograph of testes showing, sever tubular atrophy (black arrow), edema (red star) and partially atrophied tubules (yellow arrows) HandE X 100, C. Photomicrograph of diabetic Br-MSCs treated rats testes showing mild to moderate interstitial edema (red star) with normal tubules appears normal with functional spermatogenesis, spermiogenesis (yellow arrows) and hypertrophied leydig cell (red arrow), HandE X 100.
Diabetes is considered one of the most progressive metabolic syndrome sequalae that affects around 7% of people worldwide in association with various complication such as diabetic retinopathy, nephropathy, and neuropathy as well as an increased risk of limb amputation and infertility (Melendez-Ramirez et al., 2010). The results of the current study indicated that type 1 diabetes significantly reduced sperm concentration, motility and increase sperm abnormalities (Aguirre-Arias et al., 2017; Gonzales et al., 2013; Schoeller et al., 2012). This effect can be attributed to the hypoinsulinemia associated with this animal model of diabetes. Interestingly, this effect has been reported to be not only limited to the pancreatic insulin but also to testicular tissue as well as locally expressed insulin (Schoeller et al., 2012). Furthermore, testicular tissue of diabetic model showed a marked reduction in insulin level, up to 50%, compared to control rats. This reduction could adversely interfere testicular cells metabolism and energy production resulting in an eventual impact on both spermatogenesis and spermiogenesis (Schoeller et al., 2012). Our result showed that intraperitoneal infusion of Br-MSCs can ameliorate or even revert type 1 diabetes impacts on spermogram and could enhance sperm concentration, motility (Hassan and Alam, 2014; Karimaghai et al., 2018; Monsefi et al., 2013).
Diabetic hyperglycemia potentiates testicular damage with various mechanisms. Among these, local immunomodulation and testicular inflammatory state is considered more common (Donmez et al., 2014; Kushwaha and Jena, 2014; Mallidis et al., 2009). Our results were in agreement with the previous reports as type 1 diabetes elicited a significant increase in local testicular inflammatory markers (IL1β, IL6, IL8, and TGF1β) and a decrease anti-inflammatory cytokine (IL-10). Contrary to this, Br-MSCs intraperitoneal administration could ameliorate testicular inflammatory state and create a local anti-inflammatory microenvironment preventing further testicular damage. This corresponded to a significant down regulation in testicular inflammatory marker (IL1β, IL6, IL8, and TGF1β) and sharp elevation in anti-inflammatory marker (IL10) (Cakici et al., 2013; Ebrahim et al., 2018; Metwally et al., 2017). This effect can be attributed to MSCs secretion of many trophic factor that possess immunomodulatory function, eventually, decreasing the local inflammatory condition (Abd Allah et al., 2016; Hosseini et al., 2014; Kadam et al., 2016; Kadam et al., 2018; Kargar-Abarghouei et al., 2018; Twigger et al., 2013; Xing et al., 2016). These cells can significantly decrease the redox state and promote the antioxidant activity (Burova et al., 2013; Gad et al., 2017; Ghasemzadeh-Hasankolaei et al., 2016; Hassan and Alam, 2014; Sherif et al., 2018). Furthermore, these transplanted cells can progressively reduce testicular inflammatory condition and promote internal repairing mechanism via secretion of angiogenic and/or other growth factors such as insulin like growth factor, hepatocyte growth factor, vascular endothelial growth factor and fibroblast growth factors (Abd Allah et al., 2016; Hosseini et al., 2014; Kadam et al., 2018; Kargar-Abarghouei et al., 2018; Twigger et al., 2013; Xing et al., 2016).
In referring to assessment of the testicular cells proliferating capacity, mRNA expression of testicular PCNA was relatively quantified. Our result indicated that type 1 diabetes down regulated the expression of testicular PCNA (Zhao et al., 2017). This finding could be attributed to the effect of hyperglycemia that initiated testicular cellular oxidative stress and eventually potentiated a consequence of events including inflammatory process (Bornstein et al., 2004), unfolded protein response (UPR) (Herbert and Laybutt, 2016) which upregulated autophagic protein expression (Sisinni et al., 2019) and apoptosis (Zhao et al., 2017). This scenario end with a decreased cell proliferation capacity and an increased programmed cell death. In contrast, intraperitoneal administration of Br-MSCs elicited a 3-fold upregulation in mRNA expression of testicular PCNA. This effect was in agreement to previous reports (Abd El-Aziz and Metwally, 2013; Aboul Fotouh et al., 2018; Ali et al., 2017) and can be referred to the secretory activity of Br-MSCs for several trophic and growth factors (Kaingade et al., 2016). These factors stimulated the proliferation of resident stem cells initiating internal repairing mechanisms (Petri et al., 2017). Moreover, many authors attempted to activated tissue internal repairing mechanisms via reprogramming of resident stem cells through transfection of tissue with genetically engineered virus expressing many embryonic transcriptional factor (Ebina and Rossi, 2015; Hu et al., 2010; Narsinh et al., 2011).
Conclusions
The study concluded that diabetes-induced testicular dysfunction via local testicular immunomodulatory mechanism and suppressing testicular cells proliferating capacity which can be ameliorated by Br-MSCs and therefore their transplantation could present an ideal alternative strategy for therapeutic purposes.
Acknowledgments
The present study was a result of collaboration between the Departments of Pharmacology and Physiology, Faculty of Veterinary Medicine, and the Stem Cell and Molecular Biology Laboratory, Departments of Biochemistry and Pediatrics and the Medical Research Center, Faculty of Medicine at the Zagazig University, Egypt.
Authors Contribution
T.K and A.H.A conceived the project, researched data, analyzed data and drafted the manuscript. A.F.A, S.H.A, A.A.S and N.M.S researched the data and reviewed and edited the manuscript.All authors read and approved the final draft of the manuscript.
Conflict of interest
The authors declare no conflicts of interest, financial or otherwise.
References