Advances in Animal and Veterinary Sciences
Research Article
Isolation and Characterisation of E.coli Infection from the Bronchial Plug of Broiler Birds Associated with Respiratory Diseases
Sonal Vishnubhai Chaudhari1, Bholenath Pursotambhai Joshi1, Dhruv Nitinkumar Desai2*, Bharat Babubhai Bhanderi3, Komal Rameshvarlal Choudhary1, Aashwina Madhwal1
1Department of Veterinary Pathology, College of Veterinary Science and Animal husbandry, Anand Agricultural University, Anand, Gujarat; 2Immunology Section, ICAR-Indian Veterinary Research Institute, Izatnagar, Bareilly, U.P; 3Department of Veterinary Microbiology, College of Veterinary Science and Animal husbandry, Anand Agricultural University, Anand, Gujarat, India.
Abstract | The present research work was carried out to investigate the incidence of concurrent presence of Escherichia coli (E. coli) infection in broiler birds affected with respiratory distress. Detection of avian pathogenic E. coli (APEC) was performed primarily on the basis of isolation, culture and colony characteristics. Further, it was affirmatively diagnosed by PCR assaytarging four virulence genes i.e. iss, papC, tsh and vat. Antibiogram of E. coli was also conducted to estimate the prevalence of antibiotic resistance in studied poultry farm. Out of 30 tissue samples of each different poultry farms processed for bacterial isolation, a 100%prevalence of E. coli infection was found in all the 30 samples. The isolates were found highly sensitive (100%) to antibiotic disc colistin (Methane sulphonate) followed by ceftriaxone (93.33%), gentamicin (80%), levofloxacin (76.67%), co-trimoxazole (63.33%) and amoxyclav (10.00%). These results highlight the high prevelence of the bacteria and differential levels of antibiotic resistance in poultry.
Keywords |Bronchial plug, APEC, Virulence gene, Antibiogram, PCR
Editor | Kuldeep Dhama, Indian Veterinary Research Institute, Uttar Pradesh, India.
Received | July 05, 2017; Accepted | August 18, 2017; Published | August 19, 2017
*Correspondence | Dhruv Nitinkumar Desai, Immunology Section, ICAR-Indian Veterinary Research Institute, Izatnagar, Bareilly, U.P, India; Email: dhruvdesai24@gmail.com
Citation | Chaudhari SV, Joshi BP, Desai DN, Bhanderi BB, Choudhary KR, Madhwal A (2017). Isolation and characterisation of E.Coli infection from the bronchial plug of broiler birds associated with respiratory diseases. Adv. Anim. Vet. Sci. 5(8): 334-341.
DOI | http://dx.doi.org/10.17582/journal.aavs/2017/5.8.334.341
ISSN (Online) | 2307-8316; ISSN (Print) | 2309-3331
Copyright © 2017 Chaudhari et al. This is an open access article distributed under the Creative Commons Attribution License, which permits unrestricted use, distribution, and reproduction in any medium, provided the original work is properly cited.
INTRODUCTION
The etiology of respiratory disease is complex, often involving more than one pathogen at the same time (Roussan et al., 2008), which causes heavy economic losses both in terms of production and cost of treatment. Infected birds express respiratory and other lesions such as cough, respiratory distress, poor growth and production leading to high economic losses (Pang et al., 2002). Infections with the H9N2 subtype of avian influenza virus named as low pathogenic avian influenza (LPAI) have been reported frequently in many Asian countries (Ahmed et al., 2009). LPAI virus produces asymptomatic infection with variable mortality. However, when exacerbation of LPAI infection is caused by other bacterial and viral organisms or environmental conditions, severe disease with high mortality may be seen (Pazani et al., 2008). Respiratory colibacillosis is a respiratory disease caused by secondary infection with avian pathogenic E. coli (APEC). E. coli being ubiquitous organism in poultry production, any insult to the respiratory tract of chickens creates a climate for colonization of the respiratory tract. The initial insult may be viral such as infectious bronchitis (IB) or LPAI virus or bacterial such as Pasturella multocida or it may be an environmental insult such as elevated ammonia levels. The interaction of E. coli in respiratory diseases of poultry has been reviewed by Barnes and Gross, 1997. The major viral agents causing respiratory diseases in chickens are corona virus (IB), paramyxo virus (Ranikhet disease), orthomyxo virus (Avian Influenza), herpes virus (Infectious laryngotracheitis) and avian pneumo virus also called turkey rhinotracheitis (Cook and Cavanagh, 2002). The bacteria of the genus Pasteurella (P. multocida, P. gallinarum, P. haemolytica and P. anatipestifer), Bordetella (B. avium) and Haemophilus (H. paragallinarum) are involved in respiratory diseases complex (Hafez, 2002). Mycoplasma gallisepticum (MG) is also responsible for chronic respiratory disease in chickens and infectious sinusitis in turkeys (Ley, 2003). Ornithobacterium rhinotracheale has recently been identified as a pathogen causing respiratory tract infections in poultry and other birds (Chin et al., 2003).
E. coli associated with respiratory infection in chickens have also been reported (EL-Sukhon et al., 2002). Although, much is known about the individual agents responsible for respiratory diseases in poultry, uncomplicated infection with single agent is the exception. Under field condition, complicated infections involving multiple etiological agents are more commonly observed. As stated by Yashpal et al. (2004), respiratory pathogens are of major importance because they can cause disease independently, in association with each other, or in association with bacterial or viral agents.
MATERIALS AND METHODS
The study was carried out in thirty broiler flocks belongs to different broiler farms and received for post mortem diagnosis at Department of Pathology, Veterinary College, Anand. Epidemiological investigation was carried out in terms of prevalence of E.coli secondary concurrent infection in broiler farm. For isolation, identification and molecular characterization of E. coli, a total of thirty pooled samples of bronchi with plug from each farm were collected from the broiler flocks which showed gross lesions of LPAI i. e. caseous bronchitis and tracheitis.
Isolation and Identification of E. coli from tissue samples
Primary isolation and colony characteristic
The samples were collected in sterilized petri plates and triturate well to make homogenised and stored in cryovial. After that it was spread by streaking method on MacConkey agar plates. The colonies were identified by their pink colour on the culture plates. The pink coloured colonies once identified, were sub cultured on Eosin Methylene Blue (EMB) agar plates for the colony characteristic identification and simultaneously stained by gram’s staining method.
Molecular characterisation of E. coli
After isolation and colony characteristic, molecular and confirmative characterisation of E.coli was done by polymerase chain reaction method. DNA was extracted from all 30 broiler flock farm samples of bronchial tissue containing caseous plug by UltraClean® Tissue & Cells DNA Isolation kit (MO BIO, USA). The tissue was thawed and taken from each cryovial. About 50 mg of tissue was cut and used. DNA isolation was performed according to manufacturer protocol. After extraction of bacterial DNA, polymerase chain reaction was carried out for APEC virulence marker genes details were presented in Table 1. Reaction Steps and conditions of thermal cycling for different primers of virulence genes of E. coli were presented in Table 2.
Table 1: Details of primers for PCR used were adopted from Ewers et al., 2004
Primer name |
Primer sequence |
Product Size |
Gene bank Accession No. |
|
Iss |
F |
5’-CCCCAATTGGACAGAGAAAA-3’ |
174 bp |
X52665 |
R |
5’-ATCGATGGGCCTATTGTGAG-3’ |
|||
papC |
F |
5’-AATAAAAACGTGGCGGACTG-3’ |
201 bp |
Y00529 |
R |
5’-ACGCAGGTAAGCAGAATCGT-3’ |
|||
Tsh |
F |
5’-TCTCAATGCGTCGTAACAGC-3’ |
153 bp |
AF218073 |
R |
5’-CCTTCAGATGAACGTCAGCA-3’ |
|||
Vat |
F |
5’-CACGCTACTGAATGCCTGAA-3’ |
168 bp |
AY151282 |
R |
5’-TGGCAGGTTAATGGTGTGAA-3’ |
Table 2: Steps and conditions of thermal cycling for different primers of virulence genes of E. coli
Primers |
Cycling conditions |
||||
Initial denaturation |
Denaturation |
Annealing |
Extension |
Final extension |
|
iss |
940C 5 min |
940C 30 s |
560C 30 s |
720C 30 s |
720C 7 min |
Repeated for 35 cycles |
|||||
papC, vat, tsh |
940C 5 min |
940C 30 s |
580C 30 s |
720C 30 s |
|
Repeated for 35 cycles |
Antimicrobial Susceptibility Test
Furthermore, the E.coli isolates were subjected for antimicrobial susceptibility test. The antimicrobial susceptibility test was carried out by disc diffusion technique described by Bauer et al. (1966) against a panel of six antimicrobial agents. The symbols and concentrations of used antimicrobial discs are mentioned in Table 3.
Table 3: Details of antimicrobial and concentration of discs used for antimicrobial susceptibility
Sr. No. |
Name of the antimicrobial disc |
Code |
Conc. (in mcg) |
Diameter of zone of inhibition (mm) |
||
Resistant |
Intermediate |
Sensitive |
||||
1 |
Co-trimoxazole |
COT |
25 |
≤13 |
14-15 |
≥16 |
2 |
Gentamicin |
GEN |
10 |
≤12 |
13-14 |
≥15 |
3 |
Ceftriaxone |
CTR |
30 |
≤19 |
20-22 |
≥23 |
4 |
Colistin (Methane sulphonate) |
CL |
10 |
≤10 |
- |
≥11 |
5 |
Levofloxacin |
LE |
5 |
≤13 |
14-16 |
≥17 |
6 |
Amoxyclav (Amoxycillin/Clavulanic acid) |
AMC |
30 (20/10) |
≤13 |
14-17 |
≥18 |
Table 4: In vitro antibiotic sensitivity results of the E. coli isolates
Sr. No. |
Antibiotics |
Sensitivity |
Intermediates |
Resistance |
1 |
Co-trimoxazole |
(19/30) 63.33% |
(08/30) 26.67% |
(03/30) 10% |
2 |
Gentamicin |
(24/30) 80% |
(03/30) 10% |
(03/30) 10% |
3 |
Ceftriaxone |
(28/30) 93.33% |
(02/30) 6.67% |
- |
4 |
Colistin (Methane sulphonate) |
(30/30) 100% |
- |
- |
5 |
Levofloxacin |
(23/30) 76.67% |
(01/30) 3.33% |
(06/30) 20% |
6 |
Amoxyclav |
(03/30) 10 % |
(04/30) 13.33% |
(23/30) 76.67% |
RESULT AND DISCUSSION
Primary Isolation and Colony Characteristic
Primary isolation was done on MacConkey agar plate. Colonies were found pinkish colour suggesting of gram negative bacteria which was further confirmed by gram’s staining method and sub cultured on EMB agar plate as it is E.coli selective media for the isolation, it has shown greenish metallic sheen colonies. Representative of agar plates and gram’s staining were shown in Figure 1 and 2. On the basis of primary isolation and colony characteristic it was confirmed E. coli infection in all thirty poultry farm sample.
Detection of Virulence Genes in E. coli by PCR (iss, papC, tsh and vat)
APEC is diagnosed by its virulence genes that enable it to the epithelial cells is considered to be an important step in the establishment of colibacillosis. The adhesion factor is P live an extra-intestinal life. The bacterial adhesion to fim-
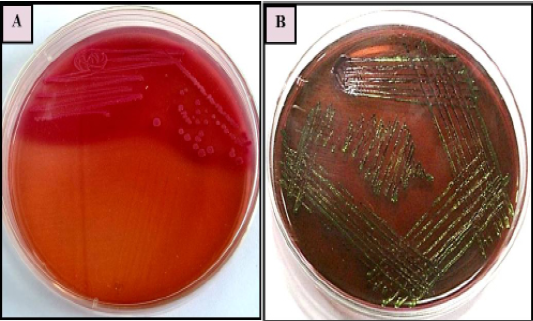
Figure 1: E. coli colonies with characteristic (A): Pink colour, lactose fermenting colonies on MacConkey agar (B) greenish metallic sheen on Eosin methylene blue (EMB) agar
bria that is coded by papC operon is located in bacterial chromosome. This factor contributes in prevention of APEC strain to be phagocytosed. iss gene contributes in increasing of APEC survival in serum and is described as an important characteristic of sever colibacillosis in broiler chickens. The temperature-sensitive haemagglutinin (tsh) gene responsible for bacterial adhesion to host cell during membrane binding process. This gene helps to development of lesions and fibrin precipitation in air sacs, increase colonization at this site and induce lesion and ulcers. vat gene responsible for vacuolating and transferring of cytotoxic produced by pathogenic.
Table 5: Prevalence of antibiotic sensitivity pattern of the E. coli isolates in the poultry farm
Farm No. |
Antibiotic sensitivity |
|||||
Co-trimoxazole |
Gentamicin |
Ceftriaxone |
Colistin (Methane sulphonate) |
Levofloxacin |
Amoxyclav |
|
1 |
R |
S |
S |
S |
S |
S |
2 |
I |
S |
S |
S |
S |
S |
3 |
I |
S |
S |
S |
S |
I |
4 |
S |
S |
S |
S |
S |
R |
5 |
I |
R |
S |
S |
S |
R |
6 |
S |
S |
S |
S |
S |
I |
7 |
S |
S |
S |
S |
S |
R |
8 |
S |
S |
S |
S |
S |
R |
9 |
S |
S |
S |
S |
S |
R |
10 |
S |
S |
S |
S |
S |
R |
11 |
S |
S |
S |
S |
S |
R |
12 |
S |
S |
I |
S |
R |
R |
13 |
S |
S |
S |
S |
S |
R |
14 |
S |
I |
S |
S |
R |
R |
15 |
S |
S |
S |
S |
S |
R |
16 |
S |
S |
S |
S |
S |
R |
17 |
I |
R |
S |
S |
R |
R |
18 |
I |
S |
S |
S |
R |
R |
19 |
R |
I |
S |
S |
R |
R |
20 |
S |
S |
I |
S |
R |
R |
21 |
S |
S |
S |
S |
I |
R |
22 |
S |
S |
S |
S |
S |
S |
23 |
S |
S |
S |
S |
S |
I |
24 |
S |
S |
S |
S |
S |
I |
25 |
I |
R |
S |
S |
S |
R |
26 |
R |
I |
S |
S |
S |
R |
27 |
S |
S |
S |
S |
S |
R |
28 |
S |
S |
S |
S |
S |
R |
29 |
I |
S |
S |
S |
S |
R |
30 |
I |
S |
S |
S |
S |
R |
DNA was extracted from tissue samples (bronchial caseous plug) and PCR has revealed that out of 30 tissue samples, 27 (90%) were found positive for the presence of iss gene followed by 25 (83.33%) for vat gene, 15 (50%) for tsh gene and 10 (33.33%) for papC gene. (Figure 4).
Among the all sample, 18 were found carrying two virulence genes out of four. There were 7 samples which were positive for three genes while 5 were having all the four virulence genes in their genome. There was no sample having only one gene of virulence during the study. Among the four different E. coli associated genes, iss gene was found more commonly associated though other genes like tsh and vat were also present to the considerable extent.
a) Detection of iss gene
Results of primer-directed amplification of the iss gene have been represented in Figure 3, which depict the presence and distribution of the amplified product. The finding of present study to detect the presence of iss gene in E. coli were similar with the findings of Ewers et al. (2004), who have found 82.7% E. coli isolates positive for the presence of iss gene from avian pathogenic E. coli. Similarly, Arabi et al. (2013) and Rocha et al. (2008) have also detected presence of iss gene in highest number of isolates i. e. 96.4% and 73.8% respectively from E. coli isolated from broiler chickens.
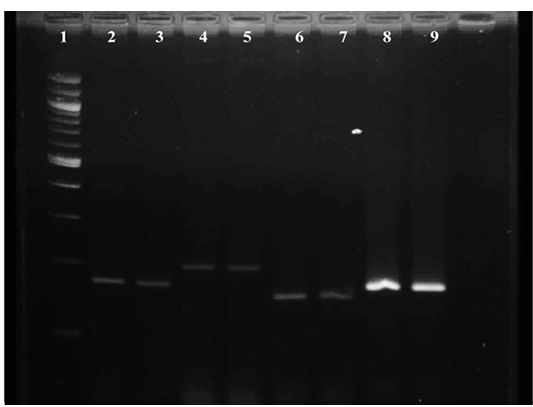
Figure 3: Gel image showing amplified product of E.coli virulence gene Lane 1 : Molecular Marker of 100 bp; Lane 2: iss +ve control; Lane 3: iss representative sample; Lane 4: papC +ve control; Lane 5: papC representative sample; Lane 6:tsh +ve control; Lane 7: tsh representative sample; Lane 8: vat +ve control; Lane 9 : vat representative sample
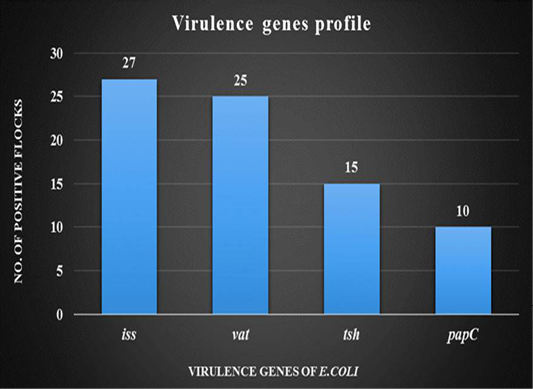
Figure 4: Graph showing virulence genes profile of thirty broiler flocks positive for Avian Pathogenic E.coli
Contrary to the present findings Won et al. (2009) reported less number of isolates (41.5%) carrying iss gene during their study for presence of virulence associated gene in E. coli. The finding of the present study i. e. detection of iss gene among 27/30 samples indicated that this gene might be common virulence gene among pathogenic E. coli in broiler chicks.
b) Detection of tsh gene
It is depicted in Figure 3, which shows the presence and distribution of the amplified product. During the study, tissue samples showed amplification with tsh gene specific primers which was to the tune of 50 per cent. The findings were in accordance with Ewers et al. (2004), Rocha et al. (2008) and Won et al. (2009) who reported presence of tsh gene in avian pathogenic E. coli as 53.3%, 55.7% and 55.9% respectively. Contrary to the present findings Arabi et al. (2013) reported highest occurrence of tsh gene among eight avian pathogenic genes, which was 96.4 per cent. Roussan et al. (2014) also detected higher presence of tsh gene to the tune of 66 per cent during their study.
c) Detection of vat gene
Representative of gene amplified product is represented in Figure 3. During the present study, E. coli from 25 tissue samples (83.33 per cent) showed positive amplification with primers specifically designed for vat gene. The findings were similar to the report of Arabi et al., (2013) and Roussan et al., (2014) who found of 85.7% and 70% occurrence of vat gene during their study whereas Ewers et al., (2004) found lower presence of vat gene 48.7% during their study.
d) Detection of papC gene
Result of primer-directed amplification of the papC gene was presented in Figure 3. During the study least number of samples were found positive for papC gene. Out of 30 tissue samples only 10 i. e. 33.33% samples were found positive for E. colipapC gene. Similarly, low occurrence (22.7%) of papC gene in avian pathogenic E. coli was observed by Ewers et al. (2004), Rocha et al. (2008) and Won et al. (2009) also found the less presence of papC gene in E. coli isolated from poultry birds which was to the tune of 24.3% and 14.4% respectively. In contrast to the present findings Roussan et al. (2014) and Arabi et al. (2013) found higher occurrence of papC which was to the tune to 50.00 and 82.10 per cent, respectively.
Molecular detection of virulence genes associated with E. coli during the present study reflected that combined effect of more than one virulence gene might be responsible for pathogenicity of E. coli organism. Among the four different E. coli associated genes, iss and vat gene were found more commonly associated though other genes like tsh and papC were also present to the considerable extent. Colisepticemia is the most important disease caused by APEC strains. This infectious disease is initiated in the avian upper respiratory tract after a primary infection caused by different virus such as the Newcasttle virus, Infectious Bronchitis virus or Mycoplasma (Gross, 1991).
These primary infections would increase the avian susceptibility to APEC strains due to the deciliation of the upper respiratory cells and exposition to ammonia and contaminated dust existing in the growth animal environment would also favour the infectious process (Oyentude et al. 1978; Nagaraja et al. 1984). Further to the virus infection, it is the initial step for colisepticemia development in birds (Gross, 1991). This infection is also referred to as aero sac disease and usually occurs among birds with 2 to 12 weeks of age, with the majority of the cases occurring among birds with 4 to 9 weeks of age with mortality reaching rates as high as 20% (Dho-Moulin and Fairbrother, 1999).
Antimicrobial Susceptibility Test
Antimicrobials have distinct advantages in the management of infection and promotion of growth in commercial broiler chicks. However, extensive use of antimicrobials indiscriminately and injudiciously could lead to emergence of antimicrobial resistance. Such uncontrolled use of drugs exerts selection pressure and promotes the proliferation of drug resistant strains of E. coli. When it is coupled with poor environmental sanitation and personal hygiene could potentially constitute a threat to public health.
In the present study, in vitro antimicrobial drug susceptibility pattern of all the thirty E. coli isolates obtained from caseous plugs in bronchi pooled samples were determined by using Disc diffusion method, as per Bauer et al. (1966). The E. coli isolates were tested against six commonly used antimicrobials. Results of in vitro antimicrobial susceptibility pattern and prevalence of antimicrobial susceptibility in poultry farm of the E. coli isolates are shown in Table 4 and 5, respectively. The isolates were highly sensitive to antibiotic disc colistin (Methane sulphonate) (100%) followed by ceftriaxone (93.33%), gentamicin (80%), levofloxacin (76.67%), co-trimoxazole (63.33%) and amoxyclav (10.00%) (Figure 5 and 6).
Sahoo et al. (2012) reported 91.43 per cent susceptibility with ceftriaxone antibiotic disc against E. coli isolates of poultry. In present study susceptibility of ceftriaxone was 93.33 per cent. Sharada and Ruben (2010) observed 55 per cent susceptibility against colistin (Methane sulphonate) antibiotic disc. In the present study susceptibility of colistin (Methane sulphonate) was found 100 per cent.
Similarly, gentamicin was also reported in susceptibility and resistance pattern by different authors, Sahoo et al. (2012), Akond et al. (2009), Hassan et al. (2014) and Atere et al. (2015) reported that gentamicin was sensitive in their study with 85.72, 87, 80, 100 and 68.8 per cent, respectively. Nasrin et al. (2012) reported that isolated bacteria were 100 per cent resistance with gentamicin. In the present study gentamicin was found 80 per cent sensitive against E.coli isolates.
Contrary to the present finding Lima-Filho et al. (2013) and Sahoo et al. (2012) observed less susceptibility of levofloxacin (51.80 %) and co-trimoxazole (28.55%) during their study from pathogenic E.coli isolates. In the present study it gave 76.67 and 63.33 per cent susceptibility respectively. Lima-Filho et al. (2013) observed 70.4 per cent resistance against amoxicillin/clavulanic acid antibiotic disc. In present study resistance of amoxyclav was 95 per cent.
The antibiotic susceptibility pattern using different antibiotic discs against E.coli isolates in poultry was reported from time to time by earlier workers. The susceptibility pattern of one antibiotic was found variable by different workers. The antibiotic susceptibility pattern of the present study showed colistin to be most sensitive antibiotic followed by ceftriaxone, gentamicin, levofloxacin and co-trimoxazole. However it should be noted that antibiotic resistance to E.coli in poultry is becoming a major issue and injudicious or unwanted use of antibiotics should be avoided and as much as possible particular antibiotic should be used after susceptibility test.
Acknowledgements
Authors are thankful to the Dean, Faculty and staff of Veterinary Pathology, Microbiology and Biotechnology of College of Veterinary Science and Animal Husbandry, AAU, Anand for their support, Cooperation and providing required facilities.
Conflict of interest
There is no conflict of interest.
Authors contribution
All the authors have contributed in terms of giving their technical knowledge to frame the article.
References
Pesa. Vet. Bras. 28(3): 183-186. https://doi.org/10.1590/S0100-736X2008000300009