Advances in Animal and Veterinary Sciences
Research Article
The Fermentation Quality and in vitro Nutrient Digestibility of Agricultural Waste Based-Complete Feed Silage with Different Composition of Oil Palm Frond and Rice Crop Residue
Budi Santoso*, Trisiwi Wahyu Widayati, Bambang Tjahyono Hariadi
Department of Animal Science, Faculty of Animal Science, University of Papua, Manokwari, West Papua 98314-Indonesia.
Abstract | The objective of the current study was to evaluate the nutritive value and fermentation quality of agricultural waste based-complete feed silage with different composition of oil palm frond and rice crop residue. Total of 4 treatments were (A) king grass 70% + cassava waste 12% + tofu waste 15% + lactic acid bacteria (LAB) 3%; (B) king grass 50% + rice crop residue 20% + cassava waste 12% + tofu waste 15% + LAB 3% (C) king grass 50% + oil palm frond 20% + cassava waste 12% + tofu waste 15% + LAB 3%; (D) king grass 50% + rice crop residue 10% + oil palm frond 10% + cassava waste 12% + tofu waste 15% + LAB 3%. The liquid of epiphytic lactic acid bacteria was sprayed on the top of silage material with a concentration of 4.0 × 106 cfu/g and then mixed by the hand. About 500 g of silage materials were packed into plastic silos and stored in the room temperature for 30 days. The results of this study showed that dry matter and organic matter contents of silage was affected (P<0.05) by different composition of oil palm frond and rice residue. Silage C had the highest dry matter and organic matter contents than other silage. There were no significant differences in crude protein and NDF contents among silage treatments with crude protein and NDF values varied from 14.5 to 15.0% and 57.1 to 58.9%, respectively. The silage A produces the highest (P<0.01) lactic acid followed by the lowest (P<0.01) pH value compared to other silage. Silages AandC had lower (P<0.01) N-NH3 concentration compared to silages B and D. Silage C had the lowest total VFA concentration compared to other silages. Silage A had the highest Fleigh Point followed by silages C, D and B. It was concluded that replacement of 20% of grass with oil palm frond in silage C produces a quality silage fermentation similar to silage A as a control.
Keywords | Agricultural waste, Fermentation, Lactic acid bacteria, Ruminant, Silage
Received | April 05, 2019; Accepted | May 25, 2019; Published | May 31, 2019
*Correspondence | Budi Santoso, Department of Animal Science, Faculty of Animal Science, University of Papua, Manokwari, West Papua 98314-Indonesia; Email: b.santoso@unipa.ac.id
Citation | Santoso B, Widayati TW, Hariadi BT (2019).The fermentation quality and in vitro nutrient digestibility of agricultural waste based-complete feed silage with different composition of oil palm frond and rice crop residue. Adv. Anim. Vet. Sci. 7(8): 621-628.
DOI | http://dx.doi.org/10.17582/journal.aavs/2019/7.8.621.628
ISSN (Online) | 2307-8316; ISSN (Print) | 2309-3331
Copyright © 2019 Santoso et al. This is an open access article distributed under the Creative Commons Attribution License, which permits unrestricted use, distribution, and reproduction in any medium, provided the original work is properly cited.
Introduction
An adequate supply of roughage to ruminants is essential for optimum rumen function and which is important to the animals health and production. However, the availability of roughage for ruminant nutrition may vary from day to day in Indonesia mainly because farmers usually own only a limited amount of land to grow forages.
The use of crop residues and agricultural wastes in animal feeding is a very common practice to ensure the feed supply for livestock production. The rice crop residue (RCR) is lower part of fresh rice crop after being harvested and left at rice field. These residues are abundantly available, and most of them are not be used as livestock feeds and is burned after dried thus causing air pollution. Santoso et al. (2011) revealed that RCR is potential to be preserved as silage and used as ruminant feeds.
The oil palm frond (OPF), a byproduct of the oil palm tree, is widely available in Manokwari regency throughout the year. Thus, the use of OPF may provide a continuous source of roughage for the ruminant livestock industry in Manokwari. In practice, the OPF is harvested periodically usually after harvesting of the fruits. Consequently, the freshly harvested OPF has to be preserved to ensure the continuity of roughage supply to the animals.
One solution to overcome the low quality of agricultural wastes is to utilize complete feed silage technology by mixing the agricultural wastes with the high quality feed sources. The provision of complete feed to beef cattle is economically more favorable compared to conventional feed (Mayulu et al., 2009), because complete silage technology can reduce the use of labor and feeding time (Baba et al., 2011). Maekawa et al. (2002) revealed that feeding the forage and concentrates separately resulted in cows consuming a high propotion of concentrates than intended, increasing the risk of ruminal acidosis (Beauchemin et al., 2002).
Forage crops can be preserved through the fermentation process of ensiling, in which epiphytic lactic acid bacteria (LAB) ferment water soluble carbohydrates (WSC) into organic acids (mainly lactic acid) in an anaerobic environment. As a result, pH is decreased and forage is preserved (Woolfrord,1990).
Lactic acid is the most commonly identified organic acid that helps reduce the pH in the silage. Therefore, LAB are the primary type of bacteria utilized in bacteria inoculants (Filya et al., 2007). Under natural circumtances, LAB grows as epiphytic bacteria, however, the population of LAB is usually low and variable with standing crops (Muck,1990). Thus, addition of LAB inoculant is needed to improve silage quality (Bureenok et al., 2006). Inoculating silages with LAB can result in a faster decrease in pH, lower final pH values, higher lactate: acetate ratios, lower ethanol and ammonia nitrogen, increase nutrient digestibility (Yahaya et al., 2004; Santoso et al., 2012).
This study was carried out to find a complete feed silage formulation based on agricultural wastes to substitute forage and evaluate their fermentation characteristic as well as in vitro nutrient digestibility.
Material and Methods
Forages Material
Rice crop residue and fresh OPF were collected from paddy and palm oil fields, respectively in Prafi District, Manokwari regency, Indonesia (longitude 133°48’E and latitude 00°53’S) with a mean altitude of 128 m sea above level. Tofu and cassava wastes were obtained from small-scale food industry located in Manokwari and Prafi Districts. In order to prepare complete silage, Tofu waste and cassava waste were dried in an oven at 60°C for at least 48 h and were ground to pass through a 1 mm sieve in Wiley mill. Rice crop residue and fresh oil palm frond were chopped into 1-2 cm lengths and immediately transfer to laboratory.
Inoculant Preparation
The epiphytic LAB inoculant was prepared according to modified of Bureenok et al. (2006) procedure as previously described by Santoso et al. (2011); Santoso et al. (2012). The inoculant was made using 220 g of fresh king grass which was macerated in 1000 ml of distilled water using a high-speed blender for 4 min. The macerated was filtered through two layers of cheese cloths and 600 ml of filtrate was collected in Erlenmeyer glass containing 18 g of glucose. The filtrate was mixed well and incubated anaerobically for 48 h at 30 ºC. At the end of 48 h, inoculant was used as source of LAB. The number of LAB inoculant was counted before the experiments by using de Man Rogosa and sharpe (MRS) which were incubated for 3 days at 35 °C (Bureenok et al., 2006).
Silage Preparation and Treatments
The fresh king grass was wilted at room temperature (approximately 28°C) for 24 h and chopped into 3-5 cm. The chopped grass was thoroughly mixed with other ingredients (Table 1). The intial LAB concentration in all inoculants were 4.0 × 106 cfu/g. About 500 g of silage materials were packed into plastic silos and stored in the room temperature for 30 days. Each treatment was prepared in triplicate.
Table 1: Composition of ingredients (%) of agricultural wastes based-complete feed silage
Experimental complete feed silage | ||||
A | B | C | D | |
King grass | 70 | 50 | 50 | 50 |
Rice crop residue | 0 | 20 | 0 | 10 |
Oil palm frond | 0 | 0 | 20 | 10 |
Cassava waste | 12 | 12 | 12 | 12 |
Tofu waste | 15 | 15 | 15 | 15 |
L. plantarum | 3 | 3 | 3 |
3 |
Preparation and Analyses of Samples
After 30 days of ensiling, samples of fresh silage were taken and dried at 60 C for 48 hours, ground to pass 1 mm screen. Dried samples were used to determine dry matter (DM), ash and crude protein (CP) according to procedure of AOAC (2005). Procedure of Van Soest et al. (1991) was used to determined concentrations of neutral detergent fiber (NDF), acid detergent fiber (ADF) and acid detergent lignin (ADL). NDF was determined without the use of a-amylase and sodium sulfite.
A 20 g of silage was macerated with 70 ml of distilled water and stored at 4oC for 24 h. It was then homogenized for 15 min by using a shaker and filtered through a Whatman No. 1542 filter paper. The filtrate was used for determine of pH,VFAs, lactic acid and NH3-N. The pH value was determined using a pH meter (Hanna Hi 9025). Concentrations of individual VFAs were analyzed using a gas chromatography (Varian CP-9002 GC, Shimadzu, Japan) equipped with flame ionization detector (FID) and stainless steel column (1500 mm × 3 mm i.d). The pressure of nitrogen was 1.25 kg/cm2. The temperature of injector oven, column oven and detector were 220,130 and 220°C, respectively. Concentrations of lactic acidand NH3-N were analyzed according to method of Barker and Summerson (1941); Chaney and Marbach (1962), respectively. Fleigh Point of the silage were calculated based on the formula used by Ozturk et al. (2006) as follows: Fleigh Point = 220 + (2 × DM% - 15) -(40 × pH), where Fleigh Point denote values between 85 and 100, very good quality; 60 and 80, good quality; 55 and 60, moderate quality; 25 and 40, satisfying quality; < 20 worthless.
In vitro Nutrient Digestibility
Determinations of DM, organic matter (OM) and NDF digestibility were conducted using modification in vitro procedure of Tilley and Terry (1963) as previously demonstrated by Santoso et al. (2018). A total of 25 ml of rumen liquor-buffer mixtures in a 1 : 4 (v/v) ratio were dispensed in 100 ml glass tubes that contained 250 mg dry sample. Triplicates of blank (with no feed sample) and standard (Pangola grass) samples were included in each run. Rumen liquor was collected in the morning prior to feeding and strained through four layers of cheese cloth into apre-warmed thermos flask. After gassing CO2 in the tube, corks were tightly placed over the tubes and were incubated in a water bath at 39°C for 48 h. After 48 h of microbial incubation, samples were incubated at 39°C for 48 h with acid-pepsin. Therefore, the contents were filtered through pre-weighed Gooch crucibles and dried at 105°C for 24 h. The percent loss in weight was determined and presented as in vitro DM digestibility (IVDMD) and in vitro NDF digestibility (IVNDFD). The remaining residue was ashed at 550°C to determine in vitro OM digestibility (IVOMD).
In Vitro Rumen Fermentation Characteristics
In vitro gas production was carried out using the Hohenheim gas test according to procedures of Menke and Steingass (1988) previously described by Santoso et al. (2018). Briefly, oven-dried samples of about 300 ± 5 mg were weighed in triplicate into 100 ml glass syringes (Model Fortune, Häberle Labortechnik, Lonsee-Ettlenschieß, Germany) with pistons that were lubricated with vaseline. The syringes were incubated in a water bath at 39°C for 24 h, before the addition of 30±1.0 ml of rumen liquor-buffer mixtures into each syringe. Each syringes were incubated in a water bath at 39°C for 48 h and were gently shaken every 8 h. The volume of gas that was released from each syringe was recorded before incubation (0 h) and 1, 2, 4, 6, 8,12, 24, 36 and 48 h of incubation. One hundred micro litter of gas was sampled from the headspace of syringe in an airtight syringe at 24 and 48 h of incubation. The gas production pattern as described in Figure 1. Methane was determined by injection 100 ml of gas into a chromatograph gas (GC model 263-50, Hitachi Ltd., Ibaraki, Japan).
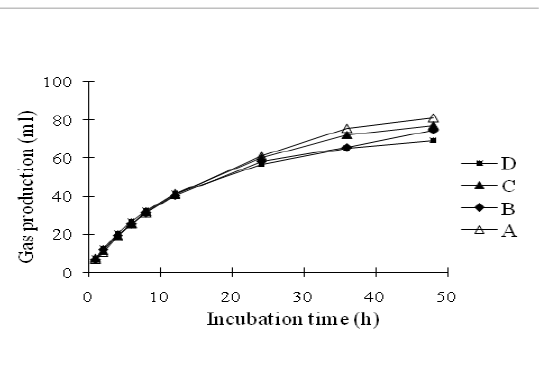
Figure 1: The pattern on in vitro gas production of agricultural waste based-complete feed silage with different composition of oil palm frond and rice crop residue
At the end of the incubation period, approximatelly 10ml of syringe contents were sampled. The pH of medium was immediately recorded using a pH digital meter (Hanna, Hi 8520, Ronchi di Villafranca, Italy). Subsequently, 0.2 ml of sub-samples were pipetted into 1.5 ml micro centrifuge tube containing 1 ml of 25 g/100 ml (w/v) metaphosphoric acid and centrifuged at 9000 × g for 10 min for volatile fatty acids (VFA) determination. For NH3-N analysis, an additional 2 ml of sub-samples were added to 2 ml of 20 g/l (w/v) NaCl.
Statistical Analysis
All data were subjected to analyses of variance of completely randomized design using general linear model (GLM) procedure of SAS 9.1 for windows (SAS Institute, 2002). The results were presented as the mean values and standard error of the means. Differences between treatment means were tested by Duncan’s multiple range test method and declared significant at P<0.05.
Results and Discussion
Characteristic of Grass Extract
After 48 h of anaerobic incubation, LAB number in king grass extract increased sharply more than 5 times and followed by decreasing pH value from 5.93 to 3.21 (Table 2). This result indicate that LAB produced high lactic acid concentration during incubation which resulted in low pH value. This result is consistent with previous studies by Santoso et al. (2009), Santoso et al. (2011) that pH value in king grass extract declined from average of 6.62 to 3.40 after 48 h of incubation at 30°C. Trend declined pH value in extracts of grass and legume after 48h of incubation have been also reported by Bureenok et al. (2006), Wang et al. (2009).
Table 2: Changes of pH value and lactic acid bacteria number in king grass extract before and after 48 h of incubation
Before incubation | After incubation | |
pH | 5.93 | 3.21 |
LAB (× 106 cfu/g) |
1.3 | 7.0 |
Physical Characteristics and Chemical Composition of Complete Feed Silages
The complete feed silage made from king grass, agricultural and food industry wastes has no fungus, acid smell and the colour was brownish green that similar with original ingredients of silage. Chemical composition of complete feed silages after 30 days ensiling are presented in Table 1. The DM content of all silages varied from 36.2 to 43.6% which was higher than the value of 30% for ideal silageas suggested by Chamberlain and Wilkinson (1996). The OM content in silage C was higher (P<0.05) than silage B.High content of OM in silage C can be due to higher OM content in OPF than RCR (Santoso and Hariadi, 2009; Santoso et al., 2016). Another reason, many organic materials are degraded to ammonia and volatile fatty acids during ensilage (Table 4). The average OM content of silages was slightly higher than value of 90.0% as reported by of Santoso et al. (2014). The NDF content was similar in all silage with an average value of 57.8 ± 0.8%. Silages A and C had lower (P<0.01) ADF content as compared to silages B and D. The high ADF value in silages B and D can be caused by fiber fraction originating from the RCR.
Fermentation Characteristics of Silages
Fermentation characteristics of the king grass silages are shown in Table 4. Parameters such as pH value, short chain fatty acids and ammonia content are commonly used as indicators fermentation quality of silage. It is generally accepted that in well preserved silages, pH values should be < 4.5 (McDonald et al., 1981) and ammonia levels <100 g/kg total N (Haigh, 1995). Silage pH is one of main factors that influence the extent of fermentation and silage quality of ensiled forage, as a low pH ensures that the forage is retained in a stable form. Silage A had the lowest (P<0.01) pH value as compared to other silage. This result is supported by high lactic acid concentration in silage A compared to other silages. Danner et al. (2003) stated that lactic acid was the strongest of all silage acids and its presence would decrease the pH more effectively than the other VFAs. In our research pH values in all silages were similar to the ideal range of 4.0 to 4.5 as was suggested by Chamberlain and Wilkinson (1996).
Silage A had the highest (P<0.01) concentration of lactic acid followed by silage C, D and B. The high concentration of lactic acid in silage A could be during the fermentation process, lactic acid bacteria ferment water soluble carbohydrates into organic acids (mainly lactic acid) in the anaerobic environment. Interestingly, the replacement of 20% of king grass with OPF in silage C resulted in a higher (P<0.01) concentration of lactic acid compared to silage B and D. However, the concentration of lactic acid in silage A and C is within the ideal range of values from 80 to 120 g/kg DM as recommended by Chamberlain and Wilkinson (1996).
Lactic acid bacteria population in silage A and C was higher (P<0.01) than silage B and D. The high population of LAB in silage A compared to other silages can be caused by the WSC content in the silage A was higher than other silages. As explained earlier that the source of forage silage A was only king grass, while silage B, C and D contain RCR, OPF or a combination of OPF and RCR. The population of lactic acid bacteria in the four silages was higher than the minimum requirements recommended by Muck (1991) that LAB populations larger than 5 log cfu/g are the minimum necessary to ensure the good fermentation of the silage.
The NH3-N is as an indicator of the proportion of the total N which has been completely degraded during ensiling (Chamberlain and Wilkinson, 1996). Therefore, concentration of ammonia-N is the best indicator of secondary fermentation. The NH3-N concentration was lower in silage without RCR and OPF (A) and silage with 20% OPF (C) than silage B and D. The low concentration of NH3-N in silage A and C was supported by the pH value of the two silages as presented in Table 4.The growth of proteolytic clostridia, which degrade protein and amino acids to NH3, is inhibited by low pH (McDonald, 1981; Winterset al., 2000). This result was consistent with previous study of Santoso et al. (2011) who found that the use of fermented forage extract increased lactic acid and greatly inhibited the clostridial activity to protect proteins from extensive degradation. Owens et al. (2002) reported that during ensiling protein is degradedto peptides and free amino acids by plant proteases. In addition, degradation of amino acids to ammonia and non-protein nitrogenous fraction is predominantly due to proteolytic clostridia. Chamberlain and Wilkinson (1996) concluded that ammonia-N is as an indicator of the proportion of the total N which has been completely degraded during ensiling. Whiter and Kung (2001) stated that homofermentative LAB reduce proteolysis and deamination of silage through a more rapid fall in pH. Concentration of NH3-N in silage A and C in this study was 82.7 and 83.4 g/kg total N that could be considered as near acceptable preserved silage according to Haigh (1995) who stated that an NH3-N <100 g/kg N usually indicates successfully preservation.
The concentrations of acetic acid, butyric acid and total VFA were affected by composition of OPF and RCR in silage. The ratio lactic acid to acetic acid in silage A, B, C and D were 7.71, 9.59, 16.77 and 9.11, respectively. Zhang et al. (2010) concluded that the ratio of lactic acid to acetic acid is a good indicator of the type of fermentation which the silage has undergone. A ratio of at least 2:1 indicates strong homolactic fermentation. Based on the ratio of lactic acid to acetic acid data, it can be seen that the highest homofermentative lactic acid bacteria was found in silage C and the lowest was in silage A.
Silage C had the lowest (P<0.05) total VFA concentration while the highest was in silage A. These results indicate that the fermentation occurs in silage C was more efficient than other silage. Chamberlain and Wilkinson (1996) stated that the VFA comprise acetic acid, propionic acid, butyric acid and other acids. The production of these acids is a reflection of an inefficient fermentation or of secondary fermentation of lactic acid to butyric acid and degradation of amino acids to ammonia with the production of amino acid from skeleton of the amino acid.
Silage A and C had higher (P<0.05) Fleigh Point than silage B. Meanwhile, the Fleigh Point of silage C was similar to silage A, suggesting that OPF can replace king grass in complete feed silage. Fleigh Point is one indicator to assess silage quality fermentation based on dry matter content and silage pH value. In addition, Fleigh Point found in the present study was higher than the value of 72.83 in alfalfa-maize silage mixture and 62.3 in rice crop residue-based silage as reported by Ozturk et al. (2006), Santoso et al. (2012), respectively.
Nutrients Digestibility
Table 5 depicts the result of in vitro DM, OM and NDF digestibilities of agricultural and food industry wastes-based silage with different composition of OPF and RCR. The in vitro DM and OM digestibility were affected (P<0.01) by composition of OPF and RCR in complete feed silage. Silage A and C had higher (P<0.01) digestibility of DM and OM on day 30 of fermentation compared with silage B and D. This result was consistent with the ADF concentration in silage A and C which was lower than silage B and D as presented in Table 3. This result was also supported by the statement of Yang et al. (2018) that the ADF content has a greater linear relationship with OM digestibility than the other parameters of nutrient digestibility.
Table 3: Chemical composition (%) of agricultural wastes-based complete feed silage with different composition of oil palm frond and rice crop residue
Complete Feed Silages | SEM |
P- value |
||||
A | B | C | D | |||
Dry matter |
36.3c |
39.0c |
43.6ab |
42.9a |
1.23 | 0.01 |
Organic matter |
92.6ab |
91.7b |
93.8a |
92.8ab |
0.37 | 0.03 |
Crude protein | 14.5 | 14.8 | 14.9 | 15.0 | 0.22 | 0.10 |
NDF | 57.6 | 57.4 | 57.1 | 58.9 | 0.81 | 0.47 |
ADF |
35.8b |
38.6a |
36.8b |
39.5a |
0.48 | 0.01 |
Hemicellulose | 21.7 | 20.6 | 18.4 | 19.3 | 0.94 |
0.14 |
Means with different superscripts in the same row differ significantly (P<0.05) and (P<0.01)
In vitro Ruminal Fermentation
The pH value, concentrations of NH3-N and VFA as well as gas production after 48 h incubation are presented in Table 6. Silage B and D had higher (P<0.05) pH value compared to silage C. The low pH value of silage A and C is a reflection of the high VFA concentration in both silages. However, the pH value in all treatments ranged from 6.60 to 6.76 which were within a range of pH (6.0 to 7.0) required for optimal rumen fibrolytic activity, as growth of fibrolytic bacteria is known to be inhibited at pH <6.0 (Weimer, 1996) and were above 6.0 which is the threshold for microbial synthesis (Russell et al., 1992). Ammonia-nitrogen concentration was not significantly different (P>0.05) between treatments complete feed silage. Availability of NH3 is an important determinant of microbial protein production as the majority of rumen bacteria use NH3 as a nitrogen source. It is important to know that concentration of NH3 will support maximal microbial growth in order to make judgments regarding utilization of non-protein N. However, the concentration of NH3-N in the present study ranged from 13.3 to 21.1 mg/dl, which was above than the NH3 concentration from 3 to 5 mg/dl as optimal to produce ruminal microorganism growth (Satter and Slyter, 1974).
Volatile fatty acids represent about 70–80% of energy absorbed by ruminants and both rates of production and proportions of individual VFAs affect energy supply as well as glucogenesis and milk production (Waghorn and Barry, 1987). Bannink et al. (2008) stated that the type of VFA formed in the rumen depends on type of substrate fermented, microbial population, and rumen environment. Concentrations of acetic acid, propionic acid, butyric acid
Table 4: Fermentation characteristics of agricultural wastes-based complete feed silage with different composition of oil palm frond and rice crop residue after 30 days of ensilage
Complete Feed Silages | SEM |
P-value |
||||
A | B | C | D | |||
pH |
4.11b |
4.89a |
4.66a |
4.89a |
0.11 | <0.01 |
Lactic acid (g/kg DM) |
84.0a |
77.3c |
80.5b |
77.5c |
0.80 | <0.01 |
Lactic acid bacteria (×106 cfu/g) |
23.1a |
2.8b |
17.7a |
5.3b |
3.30 | 0.01 |
NH3-N (g/kg total N) |
82.7b |
112.9a |
83.4b |
100.8a |
5.28 | <0.01 |
Acetic acid (g/kg DM) |
10.9a |
8.1ab |
4.8b |
8.5ab |
1.15 | 0.03 |
Propionic acid (g/kg DM) | 0.3 | 0.2 | 0.2 | 0.2 | 0.04 | 0.29 |
Butyric acid (g/kg DM) |
0.1b |
0.2b |
0.2b |
0.3a |
0.02 | <0.01 |
Total VFA (g/kg DM) |
11.4a |
8.5ab |
5.1b |
9.0ab |
1.15 | 0.03 |
Fleigh Point |
113.0a |
87.3b |
112.4a |
95.2ab |
5.86 | 0.03 |
Means with different superscripts in the same row differ significantly (P<0.05) and (P<0.01)
Table 5: In vitro nutrient digestibility of agricultural wastes based-complete feed silage with different composition of oil palm frond and rice crop residue after 30 days of ensilage
Complete Feed Silages | SEM |
P-value |
||||
A | B | C | D | |||
IVDMD (%) |
60.5b |
62.8a |
64.4a |
60.5b |
0.67 | <0.01 |
IVOMD (%) |
71.2a |
68.2b |
70.8a |
66.2b |
0.66 | <0.01 |
IVNDFD (%) | 52.4 | 51.4 | 52.6 | 51.1 | 0.56 |
0.24 |
Means with different superscripts in the same row differ significantly (P<0.01)
Table 6: In vitro fermentation characteristics in the supernatant after 48 h incubation of agricultural wastes based-complete feed silage
Complete Feed Silages | SEM |
P-value |
||||
A | B | C | D | |||
pH |
6.66ab |
6.72a |
6.60b |
6.76a |
0.03 | 0.05 |
NH3-N (mg/dl) |
19.8 | 21.1 | 19.7 | 19.3 | 0.91 | 0.54 |
Acetic acid (mM) |
48.7a |
43.6c |
45.8b |
41.2d |
0.56 | <0.01 |
Propionic acid (mM) |
18.5a |
18.5a |
19.1a |
14.3b |
0.55 | <0.01 |
Butyric acid (mM) |
9.2ab |
8.4b |
9.8a |
8.0b |
0.37 | 0.04 |
Total VFA (mM) |
76.3a |
70.4b |
74.7a |
63.5b |
1.01 | <0.01 |
Gas production (ml) |
81.0a |
74.8b |
77.2b |
69.2c |
0.88 | <0.01 |
Means with different superscripts in the same row differ significantly (P<0.05) and (P<0.01)
and total VFA were affected (P<0.05) by composition of OPF and RCR in silage. Concentration of acetic acid was significantly different (P<0.01) among silage treatments. The highest concentration of acetic acid in silage A followed by silage C, B and the lowest in silage D.Acetate is considered to be reflective of cell wall fermentation, and increased acetate levels are normally associated with declining forage quality (VanSoest, 1994). The propionic acid concentration in treatments A, B and C was similar but higher than silage D. Propionic acid concentration is associated with soluble carbohydrate ruminal fermentation (McCollum et al., 1985). Silage C had the highest (P<0.01) concentration of butyric acid, followed by silage A, B and the lowest in silage D. Silage A and C have a higher (P<0.01) total VFA concentration compared to silage B and C. This high concentration indicates that the energy produced from the fermentation of both silages were greater. The total VFA concentration in the study varied from 63.6 to 76.3 mM which is still within the normal range to support microbial growth, namely 70-150 mM as recommended by McDonald et al. (2012).
Gas production can be used as an indicator of feed degradation in the rumen. The pattern of gas production in substrate of agricultural waste and food industry wastes-based silage for 48 hours is shown in Figure 1. After 48 h incubation, silage A produced the highest (P<0.01) gas followed by silage C, B and D. This result was supported by the value of organic matter digestibility in the four silage treatments as presented in Table 5. Beuvink and Spoelstra (1992) revealed that there was a significant correlation between OM digestibility, VFA concentrations and gas production.
Conclusion
Replacement of 20% of king grass with OPF on silage C had a good fermentation quality and in vitro nutrient digestibility similar to silage A as a control. Thus, the OPF can be used as the basal feed of ruminants for forage substitution in the form of complete feed silage. However, there are needs to further in vivo studies for generalising the conclusion of this study.
AcknowledgementS
This study was financially supported by The Directorate General of Strengthening Research and Development, Ministry of Research, Technology, and Higher Education of Indonesia (contract number080/SP2H/LT/DRPM/2018). Appreciation is also extended to the The Directorate General of Strengthening Research and Development for International Conference assistance. The authors are grateful to E. M. Rohmah for the technical assistance to prepare the samples.
CONFLICT OF INTEREST
There is no conflict of interest.
AUTHORS CONTRIBUTION
All authors contributed equally.
References