Advances in Animal and Veterinary Sciences
Research Article
Evaluation of the Effects of Lipoic Acid and Spirulina platensis on Manganese-Induced Neurotoxicity in a Rat Model
Fatma Ibrahim1*, Mahmoud A. Nomier1, Mohamed A. Shaheen2, Laila M.E. Sabik1
1Department of Forensic Medicine and Clinical Toxicology, Faculty of Medicine, Zagazig University, Zagazig, Egypt; 2Department of Histology and Cell Biology, Faculty of Medicine, Zagazig University, Zagazig, Egypt.
Abstract | The aim of this study is to explore the ameliorative effects of α-lipoic acid (ALA) and/or Spirulina platensis (SP) against manganese (Mn)-induced brain damage. Sixty-four rats were classified into eight equal group, group I served as negative controls, group II received normal saline as a vehicle, group III treated with ALA (50 mg/kg/day), group IV treated with SP (300 mg/kg/day), group V treated with manganese chloride (MnCl2) at dosage of 74 mg/kg/five times/week, along with rats in groups VI (MnCl2/ALA), group VII (MnCl2/SP) and group VIII (MnCl2/ALA/SP). All treatments were administrated through oral route for eight-week, after that, the brain Mn, oxidant/antioxidant stress and inflammatory biomarkers, as well as the histopathological and immunohistochemical changes of the brain tissues were examined. Our results revealed that MnCl2 induced an overwhelming neurotoxicity evident by the elevation of brain Mn, serum protein carbonyl, brain tumor necrosis factor-α with reduction in serum superoxide dismutase and brain interleukin-10. Histologically, Mn accumulation caused structural damage, intense brain caspase-3 immuno-expression and induction of heat shock protein-70. Administration of ALA and/or SP significantly decreased the brain levels of Mn, reduce the inflammatory response, re-established the cytotoxic changes and downregulated the caspase-3 immunoexpression of HSP-70. We can conclude that simultaneous ALA and/or SP administration elicited the most favorable protective effects against the Mn-induced neurotoxicity.
Keywords | Manganese, Spirulina platensis, Lipoic acid, Neurotoxicity, Caspase-3
Received | June 04, 2020; Accepted | July 15, 2020; Published | August 10, 2020
*Correspondence | Dr. Fatma Ibrahim, Faculty of Medicine, Department of Forensic Medicine and Clinical Toxicology, Zagazig University, Zagazig, Egypt, Zip code: 44519; Email: drfatmaibrahim88@gmail.com
Citation | Ibrahim F, Nomier MA, Shaheen MA, Sabik LME (2020). Evaluation of the effects of lipoic acid and Spirulina platensis on manganese-induced neurotoxicity in a rat model. Adv. Anim. Vet. Sci. 8(10): 1034-1044.
DOI | http://dx.doi.org/10.17582/journal.aavs/2020/8.10.1034.1044
ISSN (Online) | 2307-8316; ISSN (Print) | 2309-3331
Copyright © 2020 Ibrahim et al. This is an open access article distributed under the Creative Commons Attribution License, which permits unrestricted use, distribution, and reproduction in any medium, provided the original work is properly cited.
Introduction
Manganese (Mn) is a vital nutrient for human beings, animals and plants. It is essential for normal growth, well-being and present in each living cell. In the environment, Mn exists in food, water, soil and rocks (Santamaria and Sulsky, 2010). However, Mn has an imperative biological role, the brain Mn accumulation results in severe malfunctions in the central nervous system recognized as manganism (Bouabid et al., 2016). It is a major neurotoxic complication of heavy Mn exposure, characterized by neuronal cell death and incomplete functional recovery. In an industrial setting, unprotected exposure of steel workers to Mn fumes and/or dusts can lead to fulminant neuropsychological sequences (Abbas et al., 2006). The basal ganglia is the main affected region, in particular the globus pallidus (de Moura et al., 2018).
Several theories have been postulated to explain the mechanism of Mn neurotoxicity (Erikson and Aschner, 2003). The most realistic assumption suggests that Mn has a predilection to accumulate within the brain mitochondria resulting in free radicals’ formation and neural cell death (Chtourou et al., 2011). Biologically, the balance between oxidants’ production and endogenous antioxidant defense mechanisms is warranted and any disruption for this balance results in oxidative stress damage (Tibullo et al., 2017).
Alpha lipoic acid (ALA) is a natural antioxidant, a sulfur-containing compound and it served as a necessary cofactor for a plenty of enzyme complexes (Rochette et al., 2015). It plays a protective role in biological systems owing to its disulfhydryl groups (Shila et al., 2005). Alpha lipoic acid acts through different mechanisms; i) it can antagonize the body oxidative stress status by scavenging free radicals, ii) decreasing the lipid peroxidation, iii) preventing the DNA damage caused by reactive oxygen species (ROS) along with increasing intra-cellular antioxidants (Tibullo et al., 2017).
Spirulina platensis (SP) is a multicellular cyanobacteria, has a major contribution in the health sector, food industries and aquacultures (Soni et al., 2017). Recently, SP is one of the most considerable prophylactic dietary elements because of its nutrient profile, beneficial health effects and safety profile (Serban et al., 2016). It is considered as a main dietary compound, mainly in North Africans and Mexicans due to high micro and macronutrients, e.g., antioxidant molecules, β-carotene, phycocyanin, microelements, vitamins, amino acids and fatty acids (Ku et al., 2013).
In this context, we conducted this study to explore the protective effects of ALA and/or SP against MnCl2-induced brain damage.
MATERIALS AND METHODS
Compounds
Manganese (manganese chloride tetrahydrate) was obtained from Research-Lab Fine Chemicals Industries Co., India. SP was obtained from El-hellow Co., Egypt. ALA, as Neuropatex 600mg capsules was obtained from Atos Pharmaceutics Co., Egypt.
We dissolved MnCl2, SP powder and ALA in normal saline to be orally administered to the rats.
Experimental animals
We conducted this study using 64 adult male albino rats (150 to 200 gm for each). Before launching the experiment, all animals were subjected to two-week duration of adaption to establish their physical well-being and to dismiss any diseased animals. All rats received care in agreement with the “Research Ethics Committee”, who approved the proposal of the experiment.
Study design
This study encompassed eight equal groups, with eight rats in each one.
Group I (negative control): rats received no medication; Group II (vehicle): rats were treated with normal saline (1 ml/day); Group III (ALA): rats were treated with ALA, at dose of 50 mg/kg daily according to Dwivedi et al. (2014); Group IV/SP: Rats were treated with SP, at dose of 300 mg/kg daily according to Simsek et al. (2009); Group V (MnCl2): Rats were treated with MnCl2, at dose of 74 mg/kg, five times/week (Manganese Safety Data Sheet, 2012); Group VI (MnCl2/ALA): rats were treated with ALA and MnCl2; Group VII (MnCl2/SP): rats were treated with SP and MnCl2; Group VIII (MnCl2/ALA/SP): rats were treated with ALA, SP and MnCl2 for eight weeks.
The co-treated groups received MnCl2, ALA and SP at the same formerly stated doses.
All treatments were given orally for eight weeks.
Sample collections
Blood and brain tissue collection
At the end of treatment protocol, we collected blood samples from orbital vessels of the medial canthus in clean test tubes then separation of the serum was done by blood centrifugation at 664×g for 10 minutes. The supernatant sera were stored at -20oC until assayed for the measurement of superoxide dismutase (SOD) and protein carbonyl (PC). After that, all rats were scarified by dissection and the brains were weighed. Then the brains were divided into two parts. The first one underwent homogenization then stored at -80oC to measure Mn, tumor necrosis factor-α (TNF-α) and interleukin 10 (IL-10). The other part was prepared for histopathological and immunohistochemical studies.
Brain Mn level
For digestion of the brain tissues, they were added to 5 ml of nitric/perchloric acids mixture. Then, the samples were exposed to heat at 70°C for 3 hours in a water bath, then cooling, filteration and dilution by addition of de-ionized water in order to analyze them (Zantopoulos et al., 1996). Manganese was measured by Buck scientific 210VGP Atomic Absorption Spectrophotometry at 279.5 nm wave-length.
Oxidant/antioxidant biomarkers
Superoxide dismutase was analyzed colorimetrically in accordance with the method of Nishikmi et al. (1972) at a wave-length of 560 nm, directed by the company’s guidelines (Biodiagnostic, CAT. No. SD 2521).
Cayman’s protein carbonyl assay kit (Item No.10005020). The protein-hydrozone produced was measured by spectrophotometry at an absorbance between 360-385 nm. The carbonyl content could then be a standard for protein concentration.
Inflammatory biomarkers
Tumor necrosis factor-α activity and IL-10 level were analyzed using ELISA kits (CUSABIO, CAT. No. CSB-E11987r and Mybiosource, CAT. No. MBS269138).
Histological study
Light microscopy
The brain tissues were submerged in buffered formalin 10% then consistently processed, fixed in a soft paraffin wax at 55 ºC for 2 hours and in a hard paraffin at 60 ºC for 2 h, and divided to 5 μm-thickness sections that were stained by hematoxylin and eosin (H and E) (Kiernan, 2015), then examined by light microscope.
Immunohistochemistry
The brain sections were prepared for immune-histochemical staining then incubated at 4°C with a rabbit polyclonal anti-caspase (caspase-3) primary antibody (Ab) (Ramos-Vara, 2005) or anti-heat shock protein 70 (HSP-70) primary Ab (Welch and Feramisco, 1985) in a phosphate-buffered saline (PBS). Afterwards, 3 thoroughgoing rinses with PBS, they were put in incubator with a IgG biotin-conjugated secondary Ab for 20 minutes at 32°C. Subsequently, the sections were counter-stained with hematoxylin.
Histomorphometric analysis
Morphometric analysis of sections stained with H&E, caspase-3 and HSP-70 was done by Leica Qwin 500 Image Analyzer Computer System at Pathology Department, Faculty of Dentistry, Cairo University. Vacuolated cells (%) and total average intensity of caspase-3 and HSP-70 were assessed and measured in casually-chosen 5 fields/section in different sections from each group.
Statistical analysis
The quantitative variables in different groups were stated as mean±standard deviation (SD), and were compared with each other by one-way analysis of variance (ANOVA) followed by least significant difference test (LSD). Differences were significant when the P-value (probability of chance) was <.05, while, P ≥.05 indicates a non-significant difference. All statistical comparisons were two-tailed. Vacuolated cells as percentages were assessed in H&E sections, while caspase-3 and HSP-70 were assessed as immune average signal intensity. IBM SPSS Statistics (version 24) were used for the statistical analysis.
Results
Body weight and relative brain weight
Throughout the experiment, low physical activity was remarkable in the MnCl2-treated group. Regarding the mortality rates, three rats expired in the MnCl2, MnCl2/SP and MnCl2/ALA/SP groups (individual animal from every group of them), but they were not statistically significant. MnCl2-treated group exhibited a decreased final body weight compared to the control group. ALA and/or SP caused an increased final body weight compared to MnCl2-treated group. On the other hand, the increased mean relative brain weight in MnCl2-treated group in comparison to other treated groups existed (P<.001 for each), as shown in Table 1.
Brain Mn level
We found that MnCl2-treated group revealed significant increases of brain Mn levels. The co- treatment with ALA and/or SP, resulted in significant decreases of the brain Mn level, compared to MnCl2-treated rats, as presented in Table 2.
Oxidant/antioxidant biomarkers
We observed very high statistically significant differences in MnCl2, MnCl2/ALA, MnCl2/SP and MnCl2/ALA/SP groups in comparison to the control group (P<.05). We found significant increase in mean PC with significant decrease in mean SOD activity in MnCl2-treated group in comparison to MnCl2/ALA, MnCl2/SP and MnCl2/ALA/SP treated groups, as presented in Table 2.
Inflammatory biomarkers
Very high statistically significant differences in MnCl2, MnCl2/ALA, MnCl2/SP and MnCl2/ALA/SP groups in comparison to control group were existed (P<.001).
There were significant increases in mean TNF-α and significant decreases in mean IL-10 levels in MnCl2-treated group in comparison to MnCl2/ALA, MnCl2/SP and MnCl2/ALA/SP treated groups, as presented in Table 2.
Histological study
Light microscopy
Histopathological examination of H and E-stained sections of the cerebral cortex from control group, ALA and SP treated-rats exhibited the normal histological architecture of the brain cortex. The most prominent cells in these layers were the pyramidal and granular cells along with their supporting neuroglia. The pink stained neuropils were crowded with neuronal and glial cell processes with blood vessels (Table 3, Figures 1 and 2; control).
Examination of MnCl2-treated group sections displayed marked histological abnormalities in all of the cortex’s layers with many variable sized-vacuoles between and inside most of the cells. Most of the pyramidal and granular cells were shrunken with had pkynotic nuclei and loss of their processes. The neuropils surrounding the nerve cells and neuroglia exhibited marked vacuolations (Table 3,
Table 1: Effects of MnCl2, ALA, SP and their combination on body weight and relative brain weight after 8 weeks of treatment.
Variables | Control | Vehicle | ALA | SP | MnCl2* | MnCl2 /ALA |
MnCl2 /SP* |
MnCl2/ ALA/SP* |
Initial body weight (gm) | 183.6 ±5.7 |
185.1 ±6.7 |
184.9 ±4.5 |
181.6 ±3.2 |
183.0 ±4.9 |
184.8 ±6.1 |
186.1 ±5.4 |
182.9 ±3.1 |
Final body weight (gm) | 261.4 ±5.3 |
253.2 ±7.6 |
257.5 ±4.7 |
255.7 ±7.8 |
184.4 ±6.0abcd |
228.4 ±5.9abcde |
224.6 ±4.6abcde |
240.1 ±3.6abcdefg |
Relative brain weight (gm) |
0.57 ±0.041 |
0.56 ±0.065 |
0.58 ±0.035 |
0.57 ±0.037 |
0.80 ±0.038abcd |
0.66 ±0.038abcde |
0.67 ±0.033abcde |
0.62± 0.029abcdefg |
Eight rats per group, except* seven rats. MnCl2: Manganese chloride; ALA: Alpha lipoic acid; SP: Spirulina. Data are stated as mean±SD; P value <0.05 is significant; LSD following ANOVA expressed as letters: a: significant versus control; b: significant versus vehicle; c: significant versus ALA; d: significant versus SP; e: significant versus Mn; f: significant versus Mn/ALA; g: significant versus Mn/SP.
Table 2: Effects of MnCl2, ALA, SP and their combination on laboratory findings after 8 weeks of treatment.
Variables | Control | Vehicle | ALA | SP | MnCl2* | MnCl2 /ALA |
MnCl2 /SP* |
MnCl2/ ALA/SP* |
Brain Mn (mg/dL) |
0.036 ±0.011 |
0.032 ±0.012 |
0.033 ±0.010 |
0.036 ±0.019 |
0.43 ±0.017abcd |
0.20 ±0.011abcde |
0.25 ±0.017abcdef |
0.097 ±0.020abcdefg |
SOD (U/L) |
0.286 ±0.006 |
0.285 ±0.006 |
0.282 ±0.007 |
0.289 ±0.007 |
0.154 ±0.007abcd |
0.215 ±0.007abcde |
0.241 ±0.037abcdef |
0.268 ±0.007abcdefg |
PC (nmol/mL) | 0.124 ±0.003 |
0.125 ±0.003 |
0.123 ±0.005 |
0.126 ±0.004 |
0.240 ±0.013abcd |
0.181 ±0.006abcde |
0.169 ±0.004abcdef |
0.157 ±0.005abcdefg |
TNF-α (pg/mg) |
0.139 ±0.026 |
0.158 ±0.017 |
0.148 ±0.035 |
0.140 ±0.014 |
0.343 ±0.030abcd |
0.211 ±0.012abcde |
0.199 ±0.010abcde |
0.163 ±0.008abcdefg |
IL-10 (pg/mg) |
0.268 ±0.004 |
0.266 ±0.004 |
0.265 ±0.003 |
0.269 ±0.004 |
0.140 ±0.055abcd |
0.209 ±0.007abcde |
0.225 ±0.007abcde |
0.236 ±0.005abcdef |
Eight rats per group, except* seven rats. MnCl2: Manganese chloride; ALA: Alpha lipoic acid; SP: Spirulina. Data are stated as mean±SD; P value <0.05 is significant; LSD following ANOVA expressed as letters: a: significant versus control; b: significant versus vehicle; c: significant versus ALA; d: significant versus SP; e: significant versus Mn; f: significant versus Mn/ALA; g: significant versus Mn/SP; SOD: Superoxide dismutase; PC: Protein carbonyl; TNF-α: Tumor necrosis factor-α; IL-10: Interleukin 10.
Table 3: Effects of MnCl2, ALA, SP and their combination on the histopathological findings after 8 weeks of treatment.
Variables | Control | MnCl2* | MnCl2/ALA | MnCl2/SP* | MnCl2/ALA/SP* |
Vacuolated cells (%) | 0.0±0.0 |
92.3±12.9a |
16.5±3.3ab |
16.1±3.0ab |
5.0±1.4abcd |
Caspase-3 (Total average intensity) | 4.8±0.3 |
82.6±1.4a |
7.9±0.8ab |
8.0±0.3ab |
5.8±0.5bcd |
HSP70 (Total average intensity) | 11.5±0.5 |
48.9±1.7a |
12.5±0.4b |
11.6±0.4b |
8.5±0.3 b |
Eight rats per group, except* seven rats. MnCl2: Manganese chloride; ALA: Alpha lipoic acid; SP: Spirulina. Data are stated as mean±SD; P value <0.05 is significant; LSD following ANOVA expressed as letters: a: significant versus control; b: significant versus MnCl2; c: significant versus MnCl2/ALA; d: significant versus MnCl2/SP.
Figures 1 and 2; MnCl2). Examination of sections of MnCl2-treated rats concomitantly with ALA and/or SP for timescale revealed improved nerve cells and neuroglial cells in various areas. Most of pyramidal and granular cells were more or less comparable to that of the controls. Some of the neuroglia were almost as controls, whereas scarce cells were more darkly stained and the neuropils were still vacuolated. Few pyramidal cells were less affected in between the normal granular cells (Table 3, Figures 1 and 2: MnCl2/ALA, MnCl2/SP and MnCl2/ALA/SP).
Immunohistochemistry
Immunohistochemical analysis for the detection of caspase-3 and HSP-70 in brain tissue showed intense expression of casapse-3 and HSP-70 in MnCl2 treated rats compared with other groups. Evident improvement was acquired by the use of ALA and/or SP (Table 3, Figures 3 and 4).
Histomorphometrical statistical results
We observed a significant increased percentage of the vacuolated cells with dark nuclei in MnCl2-treated group compared to control, MnCl2/ALA, MnCl2/SP and MnCl2/ALA/SP treated groups (P<0.05), (Table 3).
Similarly, a significant increase in the number of capsase-3 positive cells in MnCl2-treated group in comparison to control, MnCl2/ALA, MnCl2/SP and MnCl2/ALA/SP treated groups (P<0.05), (Table 3). Overexpression of
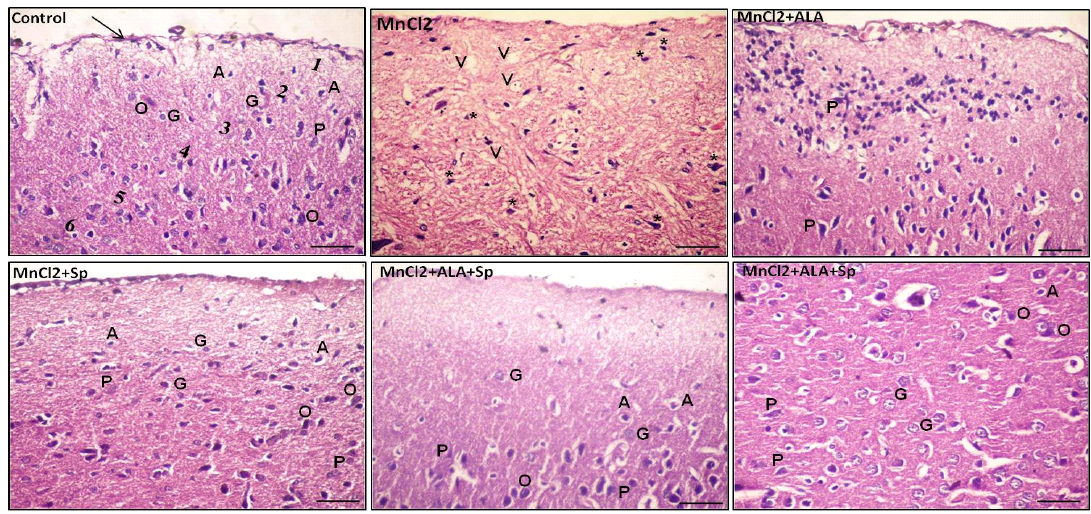
Figure 1: Photomicrograph of the rat cerebral cortex in different experimental groups. Control group consisting of pia matter (arrow), molecular layer (1), outer granular layer (2), outer pyramidal layer (3), inner granular layer (4), inner pyramidal layer (5) and multiform layer (6). MnCl2-treated group showing vaculation (V) in the neuropil. Most of the neurons and neuroglial cells had pkynotic nuclei (*) and lost their processes. The use of ALA and/or Spirulina along with MnCl2 revealed improvement in nerve cells in many areas. Pyramidal cells (P), Granular cells (G), Oligodendrocytes (O), Astrocytes (A), (Scale bar=50µm, H and E stain).

Figure 2: Photomicrograph of the rat cerebral cortex in different experimental groups. Control group with bright and vesicular nuclei (arrow) at the neuronal and neuroglial level. The neuropil is densly packed. MnCl2-treated rats presented massive vaculations (V) in the neuropil with widespread neuronal and neuroglial loss with pkynotic nuclei (*). The use of ALA and/or Spirulina along with MnCl2 showed marked enhancement in the neuropil in many areas with bright vesicular nuclei (arrow). Pyramidal cells (P) and granular cells (G), Oligodendrocytes (O), Astrocytes (A), (scale bar=20µm. H and E stain)

Figure 3: Photomicrograph of caspase-3 immunohistochemistry in the cerebral cortex showing intense expression of caspase-3 in MnCl2 treated rats compared to other groups. Marked improvement was acquired by the use of ALA and/or SP. Arrow indicates caspase-3 positive cells (stained brown) (Scale bar=50µm).

Figure 4: Photomicrograph of HSP-70 immunohistochemistry in the cerebral cortex showing increased expression of HSP-70 in MnCl2 treated rats compared to other groups. Marked improvement was acquired by the use of ALA and/or SP. Arrow indicates HSP-70 positive cells (stained brown) (Scale bar=50µm).
HSP70 in MnCl2 treated rats compared with other groups. The use of ALA and/or Sp caused marked improvement (P<0.05), (Table 3).
Discussion
Manganese is an environmental pollutant. It is incriminated as risk factor for Parkinson’s disease as well as other neurodegenerative conditions (Martinez-Finley et al., 2012).
Our study revealed that brain Mn levels were elevated with induced inflammatory and oxidative stress damage in the brain cells along with histopathological and immunohistochemical impairments. We proved that ALA and/or SP had ameliorative effects via antioxidant, anti-inflammatory and anti-apoptotic mechanisms, as well as almost restoration of brain histopathological damage caused by MnCl2. However, the most favorable protective effects against the MnCl2-induced neurotoxicity were evident with simultaneous ALA and SP administration.
Accumulation of Mn in brain is associated with a relatively long half-life and a sluggish elimination rate (Morello et al., 2008). Based on this fact, our finding showed that brain Mn levels were markedly elevated in MnCl2-treated rats. Likewise, O’Neal et al. (2014).
In our study, MnCl2 poisoning caused a significant decline in SOD activity and a significant rise in PC levels. Superoxide dismutase is a critical antioxidant in the mammalians. It has a vital role in preserving the basic cellular physiology (Fridovich, 1997; Waddington et al., 2000; Buettner et al., 2006).
Moreover, the significant low SOD activity in MnCl2-treated group could be accredited to brain mitochondrial accumulation of Mn that hinders the oxidative phosphorylation and encourages free radicals’ generation. In addition, oxidative stress can stimulate cellular membranes’ radical-mediated injury, with resultant lipid peroxidation (Deavall et al., 2012).
Oxidation of protein side chains released carbonyl groups, in particular aldehydes and ketones (Dalle-Donne et al., 2003). The carbonyl dominance in the tissues is a reflection of Mn ability to overproduce reactive free radicals overwhelming the antioxidant system capacity (Liu et al., 2013).
Our findings established that MnCl2 exposure for eight weeks was associated with significant upregulation of brain TNF-α levels with significant downregulation of IL-10. Adipocytes and the peripheral tissues are the key producers of TNF-α as well as they augment tissue‐specific inflammatory cascades mediated by ROS and activation of different transcriptional pathways (Akash et al., 2018).
In corroboration, Nadeem et al. (2018) and Nkpaa et al. (2019), clarified the inflammatory signaling of Mn induced neurotoxicity. Furthermore, Mn stimulates lipopolysaccharide‐induced microglial cytokines production such as TNF‐α (Filipov et al., 2005; Liu et al., 2009).
Interleukin-10 is a potent anti-inflammatory cytokine that is expressed by innate/adaptive immune cells and the injurious agent. It acts by inhibition of the immune reaction of macrophages and monocytes (Moore et al., 2001; Mosser, 2003; Saraiva and O’Garra, 2010). In this vein, Song and Coworkers (2016), stated that exposure to Mn led to downregulation of IL-10 levels.
Histopathological findings in this study coincide with Chtourou et al. (2010); Adedara et al. (2017). These findings are linked to oxidative damage in the brain with subsequent reduction in antioxidant defenses with accumulation of ROS following prolonged Mn exposure.
Immunohistochemical examination of brain sections of all MnCl2-treated rats in the current work showed many neurons with strong positive reactions to the caspase-3 antibodies in their cytoplasm. These findings agree with Nadeem et al. (2018), who found that Mn caused increased caspase-3 expression to about 11-fold as in comparison to the controls.
Intense positive reaction to caspase-3 in the present work could be accredited to the exaggerated oxidative stress that triggered instabilities in the permeability of mitochondrial membranes and free radicals escape of from the mitochondria to the cytosol, which attach to apoptotic protease activating factor 1 (Apaf-1), and trigger the caspase cascade, resulting in cell death (Liu et al., 2005).
Our results disclosed that MnCl2 elicited strong positive HSP-70 reaction. These findings coincide with preceding researches, which stated that Mn exposure modifies the HSPs expression in different species. Many evidences refer to increased HSP-70 levels after Mn exposure, as a trial for protection from the toxic and oxidative stress effects elicited by Mn (Zhang et al., 2002; Zhu et al., 2013; Nicosia et al., 2015). Positive staining for HSP-70 in the brain tissue mirrored the oxidant overhelming and the exhaustion of the antioxidant enzymes (Kalmar and Greensmith, 2009).
“Antioxidants” term could be applied to minerals, vitamins or amino acids. Antioxidants may act separately or collaborate defending each other on exposure to oxidative stress. Furthermore, every single antioxidant is extremely definite for one or two free radicals (Tibullo et al., 2017).
Alpha lipoic acid or its reduced active form, Dihydrolipoic acid (DHLA) have a multitude of biochemical-biological functions like regeneration endogenous antioxidants for example, ascorbic acid vitamin E (Rochette et al., 2013). In addition, ALA and DHLA act as direct ROS scavengers, and could bind redox-active metal complexes, as free iron, copper and Mn (Holmquist et al., 2007; Gorąca et al., 2011).
In our study, co-administration of ALA resulted in decreased brain Mn, PC and TNF-α, besides increased SOD activity and IL-10 levels. Moreover, ALA improved the histopathological and immunohistochemical changes induced by MnCl2.
Our results agree with the previous study of Luo et al. (2017), which stated that ALA increased the body weight gain when co-administrated with toxic metals (Luo et al., 2017). According to Moini et al. (2002), ALA acts as an insulin sensitizer that improves insulin sensitivity and hastens the recovery of pancreatic β-cells improving the glucose uptake.
Thiols provide safeguard against lipid peroxidation in biological systems (Stankiewicz et al., 2002). Alpha lipoic acid, a dithiol, scavenges the free radicals as well as chelates the free iron involved in these radicals formation (Packer et al., 1997). Additionally, ALA and DHLA can cross freely the blood brain barrier and cell membranes, promoting the activation of other antioxidants molecules as coenzyme Q10 (Mihai et al., 2010).
Alpha lipoic acid supplementation decreased the inflammatory TNF-α and increased anti-inflammatory IL-10 levels according to Zembron-Lacny et al. (2013). Besides, the capability of ALA to inhibit the inflammatory mediators is based on nuclear factor-kappa B activation (Tanaka et al., 2015).
In this study, SP antagonized the MnCl2-induced neurotoxicity; as indicated by decreased brain Mn, PC and TNF-α, as well as increased SOD and IL-10. Additionally, SP reversed the histopathological and immunohistochemical changes induced by MnCl2.
Concerning the body weight, our results agree with other studies which confirmed that co-treatment with SP significantly increased the body weight as compared to rats exposed to toxic agent only (Ponce-Canchihuaman et al., 2010; Gaurav et al., 2011).
The gastrointestinal tract, the first contact site of the body to various xenobiotics administered orally, is rich in cytokines, growth factors and humoral mediators that control the activity of the liver, the pancreas and the GIT-brain neurohumoral axis. In this context, the oral route of SP administration had great benefits to overcome Mn poisoning (Strader and Woods, 2005).
According to Gelagutashvili et al. (2003); Bashandy et al. (2016); Khalil et al. (2018); SP can act as metal chelator that can eliminate Mn from the body. The protective role of SP could be accredited to its unique composition of antioxidants, such as C-phycocyanin, carotenes, microelements, amino acids, fatty acids and carbohydrates which have influential antioxidant and anti-inflammatory properties (Abdelkhalek et al., 2015).
In our study, SP showed better protective effect than ALA. However, the combined supplementation effectively caused marked improvement superior to the solitary use of each. That is most likely because of the combined antioxidant, anti-inflammatory effects, along with neuroprotection of both.
Conclusion
We found that MnCl2 exposure induced evident damage in brain tissue in rats, as determined by biochemical and histological alterations. The administration of ALA and/or SP protected against MnCl2-induced neurotoxic effects. The most desirable protective effect against the MnCl2-induced neurotoxicity was achieved in combined ALA and SP supplementation.
Acknowledgement
The author would like to thank Rofaida Ashraf Afifi (British medical student) for her great help with the manuscript English language editing.
Authors Contribution
Mahmoud A. Nomier and Laila M.E. Sabik planned this study and revised the manuscript. Fatma Ibrahim performed the experimental work, samples collection and all laboratory tests. Mohamed A. Shaheen performed the histological studies. All authors shared manuscript writing.
Conflict of interest
The authors have declared no conflict of interest.
References