Journal of Animal Health and Production
Research Article
Ameliorative Effect of Azolla pinnata on Imidacloprid Induced Hepatorenal Toxicity, Oxidative Stress and Immunosuppression In Nile tilapia
Asmaa A. Attia1*, Hamad A. El-Saadawy2, Hussein I. El-Belbasi2, Samah A.A. Abd El-Hameed1
1Fish Health and Management Department, Central Lab for Aquaculture Research, Abbassa, Abu Hammad, Sharkia, Egypt; 2Biochemistry Department, Faculty of Veterinary Medicine, Zagazig University, Sharkia, Egypt.
Abstract | The current study aimed to investigate the protective impact of dietary supplementation of Azolla pinnata against sublethal toxicity of Imidacloprid (IMI) in Nile Tilapia. A total of 180 fish were distributed randomly to six groups in triplicate. The first group (control) was fed a basal diet, the second (A 2%) and third groups (A 4%) were fed on basal diets supplemented by 20 g kg−1 and 40 g kg−1 of diet Azolla, respectively. However, the fourth, fifth, and sixth groups, exposed to 0.05 μgL−1 IMI (1/2 LC50), were fed supplemented diets as follows: IMI (basal diet), IMI-A 2% (Azolla, 20 g kg−1), IMI-A 4% (Azolla, 40 g kg−1) respectively, for 8 weeks. The findings showed that a significant (p˂0.05) elevation in the levels of serum alanine and aspartate aminotransferases (ALT and AST), alkaline phosphatase (ALP), urea, creatinine, and malondialdehyde (MDA), meanwhile, the antioxidant enzyme activities (SOD, CAT, and GPx), reduced glutathione (GSH) content, nitric oxide (NO) as well as lysozyme activity were markedly (p˂0.05) declined in the IMI-exposed fish. Interestingly, Azolla supplementation attenuated these variables with improving liver and kidney markers (p˂0.05). Therefore, Azolla pinnata may be a valuable candidate as a natural antioxidant for ameliorating imidacloprid toxicity in Nile tilapia.
Keywords | Azolla pinnata, Imidacloprid, Immunoresponse, Hepatorenal functions, Oxidative stress, Nile tilapia.
Received | December 29, 2020; Accepted | January 19, 2021; Published | March 25, 2021
*Correspondence | Asmaa A. Attia, Central Lab for Aquaculture Research, Abbassa, Abu Hammad, Sharkia, Egypt; Email: asmaaattia@hotmail.com
Citation | Attia AA, El-Saadawy HA, El-Belbasi HI, Abd El-Hameed SAA (2021). Ameliorative effect of azolla pinnata on imidacloprid induced hepatorenal toxicity, oxidative stress and immunosuppression in nile tilapia. J. Anim. Health Prod. 9(s1): 1-6.
DOI | http://dx.doi.org/10.17582/journal.jahp/2021/9.s1.1.6
ISSN | 2308-2801
Copyright © 2021 Attia et al. This is an open access article distributed under the Creative Commons Attribution License, which permits unrestricted use, distribution, and reproduction in any medium, provided the original work is properly cited.
Introduction
Imidacloprid (IMI), a new generation of neonicotinoid insecticides, has a broad potential spread because of its agonistic effect on insects over vertebrates (Buszewski et al., 2019). From 2013 to 2015, the European Commission prohibited the use of three neonicotinoid pesticides including imidacloprid (Baroso, 2013). Because of their bioaccumulation in fish, insecticides are extremely dangerous to fish and other organisms in the food chain (Bojarski and Witeska, 2020). Fish is one of the non-target organisms that could be affected by pesticides once releasing to the environment (Ojesanmi et al., 2017).
Nile tilapia, one of the most common freshwater fish species in Egypt and worldwide, was considered to be ideal in laboratory studies because of its various benefits, such as high growth rate and reproductive levels, laboratory resistance and tolerance and commercial food adaptation (Ghozlan et al., 2017). Despite exposure to imidacloprid residues, the potential for toxicity, persistence, and bioavailability may be significantly decrease but has led to stress in the ecosystem (Van der Sluijs et al., 2013).
Azolla pinnata, an aquatic free-floating fern, is a promising food because of its good nutritional value (Das et al., 2018). Azolla consists of a higher crude protein and essential amino acid (rich in lysine), besides Phyto-constituents such as alkaloids, flavonoids, phenols, tannins, and saponins (Selvaraj et al., 2013). The efficacy of both flavonoids and many other phenolic components have been documented as antioxidants, anti-inflammation, and immune system promoting (Tungmunnithum et al., 2018). Furthermore, it contains certain macronutrients like calcium, phosphorus, potassium, and other minerals in trace levels (Anitha et al., 2016). Azolla has an immune potential effect owing to high content of carotene (Dhumal et al., 2009) besides, essential amino acids, vitamins and trace elements (zinc , copper, iron, and selenium) (Calder and Kew, 2002).
Because of its high phenolic and flavonoid content, the application of Azolla pinnata as an antioxidant and immunostimulant agent has been suggested in complementary and alternative medicines (Nayak et al., 2015). Consequently, the current study was performed in order to assess the potential protective impact of Azolla pinnata on biochemical, antioxidant, and immunological parameters in Nile tilapia against sublethal effects of IMI.
Materials and Methods
Fish
Nile tilapia (n = 288; 20.22 ± 0.5 g, initial weights) were obtained from Central Laboratory for Aquaculture Research (CLAR) ponds, Sharqia, Egypt. Fish were moved to the wet laboratory and placed there for acclimation to the laboratory conditions for 2 weeks. The aquaria, filled with dechlorinated, and fresh tap water, were continuously aerated by an electric pump, and the temperature was held at 27.8 ± 0.1 °C by heaters. Water samples were obtained, from each aquarium, weekly to monitor various water quality parameters. According to Boyd and Tucker (2012), the mean values of water parameters were within the acceptable ranges (dissolved oxygen 8.21 ± 0.12 mg/l, pH 8.39, and unionized ammonia concentration 0.15 ± 0.01 mg/l). During the acclimation period, fish were fed on a basal diet twice daily. The experimental protocols were followed by Ethics of Animal Use in Research Committee, Faculty of Veterinary Medicine, Zagazig University, Egypt.
Diet preparation
A Basal diet was a commercial fish diet (ALLER AQUA, Denmark) containing 30 % crude protein, 5 % crude lipids, and 4 % fiber. For preparing the experimental diets, Azolla powder was prepared in CLAR. Briefly, Fresh Azolla pinnata (A), obtained from Agriculture Research Center, were sundried and finely ground. Two tested diets were prepared whereas Azolla powder was added in a blender with basal diet at a dose of 20 g kg−1 diet and 40 g kg−1 diet, mixed thoroughly, pelletized, and dried air for 24 hours at room temperature after that preserved at 4 °C until used.
Lethal concentration bioassay (LC50 - 96 h)
Fish were exposed to IMI (C9H10C1N5O2) which was purchased as CLOPRID plus 35% SC produced by European Community for agricultural products, Germany. Fish were divided into 18 glass aquaria (n = 108, 6 fish/aquarium, triplicates, 6 groups). The first group (control group) was exposed to dechlorinated tap water without the IMI, while the other test groups were exposed to various concentrations of IMI dissolved in distilled water ranging from 50 to 250 μgL−1 for 96 h. During this experimental period, Fish have not been fed and the mortality rates were reported at 24, 48, 72, and 96 h interval. The dead fish were immediately removed from the aquaria. As shown in Table 1, The LC50 value for 96 h was determined using Behrens and Kärber (1935) method (LC50 = highest dose − ∑ (a × b)/n)
Experimental Design
After acclimation, 180 fish were randomly allocated into 18 glass aquaria (10 fish/aquarium, triplicates, 6 groups). Group1, Control, was fed a basal diet. Group 2, A (2%), was fed A-supplemented diet at 2% (Azolla, 20 g kg−1). Group 3, A 4%, was fed A-supplemented diet at 4% (Azolla, 40 g kg−1). Group 4, IMI, exposed to ½ 96 h-LC50 of IMI (0.05 μgL−1), was fed a basal diet. Group 5, IMI-A 2%, exposed to ½ 96 h-LC50 of IMI (0.05 μgL−1), was fed A-supplemented diet at 2%. Group 6, IMI-A 4%, exposed to ½ 96 h-LC50 of IMI (0.05 μgL−1), was fed A-supplemented diet at 4%. The experiment for all groups was continued for 8 weeks.
Sampling
At the end of the fourth and eighth weeks, fifteen fish from each group were anesthetized with MS-222 (100 μg ml−1) and samples of blood were collected from the caudal vein without anticoagulants and left to clot at room temperature. Serum has been isolated by centrifugation at 3000 xg for 15 min and then preserved at −20 °C ever since analysis. Fish were sacrificed by cervical dislocation quickly after blood sampling then the liver was removed and immediately rinsed in cold physiological saline (NaCl 0.9 %) for oxidative stress analysis.
Serum biochemical assay
Serum transaminases (ALT and AST), alkaline phosphatase (ALP), urea, and creatinine have been estimated by using kits (Biodiagnostic Co., Giza, Egypt) according to the manufacturer instructions.
Hepatic oxidative stress markers
Malondialdehyde (MDA) level, one of the end products of lipid peroxidation, was assayed according to Ohkawa et al. (1979). Superoxide dismutase (SOD) activity was analyzed following Nishikimi et al. (1972) procedure. Catalase (CAT) activity was determined following Aebi (1984)
Table 1: Lethal concentration (LC50 – 96 h) for IMI in Nile Tilapia (n = 6)
Group | IMI conc. (μgL−1) | No. of Dead fish after 96 hr | a | b | a*b |
1 | 0 | 0 | 50 | 0 | 0 |
2 | 50 | 2 | 50 | 1 | 50 |
3 | 100 | 3 | 50 | 2.5 | 125 |
4 | 150 | 4 | 50 | 3.5 | 175 |
5 | 200 | 5 | 50 | 4.5 | 225 |
6 | 250 | 6 | 50 | 5.5 | 275 |
∑ | 850 |
Difference in dose between two successive concentrations (a), mean of dead fish in the groups (b)
Table 2: Effect of dietary Azolla pinnata supplementation on serum biochemical parameters of Nile Tilapia influenced by sublethal IMI-polluted water for 8 weeks.
Parameters | Experimental groups | |||||
Control | A (2%) | A (4%) | IMI | IMI-A (2%) | IMI-A (4%) | |
ALT (U/L) | ||||||
4 weeks |
30.15±0.66c |
29.63±0.37c |
29.14±0.60c |
54.24±0.6a |
43.20±0.6b |
41.60±0.8b |
8 weeks |
31.24±1.01bc |
30.53±0.77c |
29.82±0.25c |
67.20±0.6a |
35.27±0.63b |
32.40±0.23c |
AST (U/L) | ||||||
4 weeks |
26.12±0.65d |
27.53±0.32d |
27.11±1.09d |
45.29±1.05a |
36.27±0.75b |
32.16±0.9c |
8 weeks |
26.60±0.34c |
28.21±0.69c |
26.33±0.77c |
59.14±2.39a |
32.16±0.72b |
28.64±0.37bc |
ALP (U/L) | ||||||
4 weeks |
6.40±0.23c |
6.53±0.13c |
6.30±0.17c |
11.21±0.2a |
8.73±0.32b |
8.20±0.29b |
8 weeks |
6.61±0.28b |
6.80±0.34b |
6.47±0.21b |
14.18±1.30a |
7.55±0.31b |
7.29±0.37b |
Urea (mg/dl) | ||||||
4 weeks |
3.12±0.06c |
3.11±0.06c |
3.10±0.11c |
6.15±0.08a |
4.42±0.11b |
4.12±0.16b |
8 weeks |
4.22±0.04b |
4.25±0.14b |
4.21±0.12b |
8.65±0.26a |
4.73±0.32b |
4.55±0.20b |
Creatinine (mg/dl) | ||||||
4 weeks |
0.54±0.01c |
0.56±0.01c |
0.55±0.01c |
1.27±0.04a |
0.72±0.02b |
0.67±0.03b |
8 weeks |
0.56±0.01b |
0.59±0.01b |
0.57±0.01b |
1.80±0.12a |
0.63±0.02b |
0.60±0.01b |
Values (means ± SE) with different superscripts are significantly (p˂0.05) different in the same row. Azolla pinnata (A), (IMI), alanine aminotransferase (ALT), aspartate aminotransferase (AST), alkaline phosphatase (ALP)
method. Glutathione peroxidase (GPx) activity was measured following Paglia and Valentine (1967) method. Reduced glutathione (GSH) was done according to Beutler et al. (1963).
Serum immunological assay
Nitric oxide (NO) was estimated calorimetrically according to the method of Montgomery and Dymock (1961). The serum lysozyme activity was assessed using turbidity measurement as mentioned by Schäperclaus (1992).
Statistical analysis
The variance (mean ± Standard error) was analyzed by one-way analysis of variance (ANOVA) followed by Duncan’s multiple range test (DMRT) by using SPSS Version 25 and Differences are considered significant when p<0.05.
Results
Hepatorenal markers
Table (2) demonstrates A. pinnata’s improving role in combating the sub-lethal effect of IMI on hepatic and renal function of Nile tilapia. Enzymatic activities of ALT, AST, and ALP in IMI exposed fish were significantly (p˂0.05) enhanced compared to the other groups. Furthermore, serum urea and creatinine were significantly (p˂0.05) higher in IMI exposed fish versus the other groups. On the contrary, Azolla significantly (p˂0.05) declined the damage in liver and kidney of combined treatment with IMI, particularly IMI-A 4% compared to IMI and IMI-A 2%. Regarding Azolla treated groups, a non-significant change (p˃0.05) was observed in the studied parameters between Azolla (A 2% and A 4%) and control groups.
Table 3: Effect of dietary Azolla pinnata supplementation on immune responses of Nile Tilapia influenced by sublethal IMI-polluted water for 8 weeks.
Experimental groups | ||||||
Control | A (2%) | A (4%) | IMI | IMI-A (2%) | IMI-A (4%) | |
NO (μmol L −1) |
||||||
4 weeks |
43.30±0.43f |
58.30±0.49d |
61.40±1.10c |
55.16±0.25e |
66.70±0.40b |
70.21±0.77a |
8 weeks |
45.20±0.35c |
62.81±0.39b |
67.25±1.01a |
17.40±0.23f |
39.80±0.81e |
43.20±0.38d |
Lysozyme (unit/mg protein) | ||||||
4 weeks |
3.56±0.03d |
4.26±0.13c |
4.55±0.25bc |
4.12±0.07c |
4.94±0.08b |
5.50±0.12a |
8 weeks |
3.58±0.08b |
4.64±0.09a |
4.79±0.11a |
1.25±0.06c |
3.42±0.05b |
3.54±0.08b |
Values (means ± SE) with different superscripts are significantly (p˂0.05) different in the same row. Azolla pinnata (A), Imidacloprid (IMI), Nitric oxide (NO).
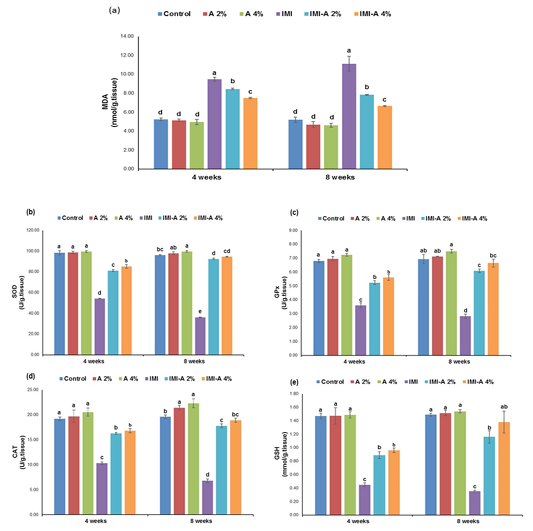
Figure 1: Effect of dietary Azolla pinnata supplementation on hepatic oxidative stress markers of Nile Tilapia influenced by sublethal IMI-polluted water for 8 weeks. Values (means ± SE) with different superscripts are significantly (p˂0.05) different in the same row. Azolla pinnata (A), imidacloprid (IMI), (a) Malondialdehyde (MDA), (b) Superoxide dismutase (SOD), (c) Glutathione peroxidase (GPx), (d) Catalase (CAT), (e) Reduced Glutathione (GSH)Section of intestine showing sloughing of villli and heterophillic infiltration)
Hepatic oxidative stress markers
MDA concentration in hepatic tissue of Nile tilapia, exposed to IMI, was significantly (p˂0.05) increased versus the other experimental groups. However, IMI-A 2% and IMI-A 4% efficiently diminished MDA level, even though it couldn’t meet control and Azolla (2 % and 4%) levels (Figure 1a). A-supplemented diet (2 % and 4 %) showed insignificant (p˃0.05) reduced MDA levels versus the control group. Concerning antioxidant status, IMI exposed fish and the preventive role of Azolla are presented in Figure 1 (b-e). Antioxidant enzyme activities, SOD, CAT, and GPx, and GSH content in IMI treated group were significantly (p˂0.05) declined as regards the other experimental groups. However, a significant (p˂0.05) rise in SOD, CAT, GPx, and GSH concentration between in IMI-A 2% and IMI-A 4% was observed in a concentration-dependent manner.
Serum immunological parameters
In Table (3), the increase in the levels of serum NO, and lysozyme activity at 4 weeks was significant (p˂0.05) in all fish treated groups comparing with the control group. After 8 weeks, a significant (p˂0.05) decline in the levels of serum NO, and lysozyme activity in IMI exposed fish were observed, meanwhile, these parameters were significantly boosted (p<0.05) in IMI-exposed fish fed on A-supplemented diet (2 % and 4 %).
Discussion
The purpose of current work was to explore the sublethal effects of Imidacloprid in Nile tilapia and the potential prevention of these by A. pinnata. The liver plays a major role in metabolism and carries out vital functions such as detoxification. In the present study, the hepatic biochemical assay, ALT, AST, and ALP, showed a significant increase in IMI-exposed group compared to the other groups. Similarly, elevated levels of these enzymes have previously been recorded from IMI exposure (Mahajan et al., 2018) or other pesticides (Hussein et al., 2019). These enhanced values indicate that the liver tissues are severely damaged resulting from oxidative stress induced by IMI exposure. The generation of free radicals from IMI toxicity interacts with the cellular membrane, causing lipid peroxidation and alternations in membrane fluidity, leading to leakage of these biomarkers into the blood (Mahajan et al., 2018). On the other hand, dietary supplementation of Azolla attenuated IMI-induced hepatotoxicity. The protective effect of Azolla can be triggered by the indirect stabilization properties of membrane, such as all the polyphenols (Gupta et al., 2016).
Serum urea and creatinine increased values in IMI-exposed fish have been shown as indicator of renal dysfunction. Similar results were reported from fish exposed to IMI (Qadir et al., 2014). These findings may be related to imidacloprid reducing effect on glomerular filtration and protein catabolic rates which may in turn lower the kidney’s capacity to eliminate urea and creatinine (Qadir and Iqbal, 2016). However, dietary supplementation of Azolla alleviated IMI-induced nephrotoxicity confirmed by lowering urea and creatinine levels. Similar to this observation, Abdelkhalek et al. (2017) in Nile tilapia.
Reactive oxygen species are usually produced during mitochondrial oxidative metabolism and cellular xenobiotics response (Ray et al., 2012). A significant elevation of MDA level in IMI- exposed fish liver, along with a dramatic decline in antioxidant enzyme activities, SOD, CAT, and GPx, and GSH content as compared to the other groups, was noted in this study. It indicated that IMI exposure leads to excessive oxidative stress, so the antioxidant enzymes were unable to eliminate that stress and were exhausted (Vieira et al., 2018). The increase of MDA level is an indication of IMI-induced lipid peroxidation leading to tissue injury. These results are in accordance with Mahajan et al. (2018) showed an increase in MDA level, while a significant decrease in antioxidant enzyme activities following repeated oral administration of IMI in liver of rats. Moreover, a significant decline of GSH content in IMI exposed fish liver is most probably due to the consumption of GSH. Similar results of the study carried out by (Naiel et al., 2020). The supplementation of Azolla at 2% and 4% was effective in reducing hepatic oxidative damage as indicated by the assessment of antioxidants. Our findings reported that Azolla supplementation reduces oxidative stress in fish liver, as demonstrated by higher antioxidant enzyme activities, SOD, GPx, and CAT, along with reduction of lipid peroxidation product (MDA). Improving the antioxidant status could be linked to the antioxidant strength of A. pinnata through reducing the accumulation of free radicals produced during IMI-induced peroxidation of lipid (Nayak et al., 2015). In addition, it can be clarified that Azolla can scavenge free radicals circulating and increase the expression of antioxidant enzymes at transcriptional levels, such as most of biophenols (Huyut et al., 2017).
Nitric oxide and lysozyme are used as an indicator for nonspecific immune functions in fish (Abd El-Gawad et al., 2016). In the present study, IMI exposure induced immunological alterations in Nile tilapia, such as an increase in serum level of NO and lysozyme activity in IMI-exposed group after 4 weeks, however, at the end of 8 weeks, the level of No and lysozyme activity declined as compared to the other groups. This alteration in the IMI-exposed group may be attributed to immune suppression that were detected in fish at a sub-lethal level (Werner and Oram, 2008). Similar to this observation, Rahman et al. (2020) in common carp found marked reductions in the levels of No and lysozyme activity after exposure to profenofos (47.46 μgL−1). On the other hand, A. pinnata improved the immune suppression induced by imidacloprid. This improvement demonstrated that the antioxidant potential of A. pinnata could maintain the functional and structural integrity of important immune cells and in turn enhance immune response as reported by Mounes et al. (2020).
Conclusions
The present study indicated that the supplementation of Azolla at 2 % and 4 % for 8 weeks to Nile Tilapia protect liver and kidney from IMI toxicity by enhancing oxidative responses, serum biochemical and immunological activities; thereby protecting the liver tissue integrity.
conflict of interest
The authors have declared no conflict of interest.
Authors contribution
All authors contributed equally to this work.
References