The Journal of Advances in Parasitology
Research Article
Ontogeny of Intestinal Permeability in Chickens Infected with Eimeria Maxima: Implications for Intestinal Health
Gustavo H. Schneiders1, James C. Foutz1, Marie C. Milfort1, Ahmed F. A. Ghareeb1, Ufuoma G. Sorhue1,2, Jennifer N. Richter1, Alberta L. Fuller1, Susan M. Williams3, Romdhane Rekaya4,5, Samuel E. Aggrey1,5*
1Nutri Genomics Laboratory, Department of Poultry Science, University of Georgia, Athens, GA 30602, USA; 2Delta State College of Education, Mosogar, Sapele, Nigeria; 3Department of Population Health, University of Georgia, Athens, GA 30602; 4Department of Animal and Dairy Science, University of Georgia, Athens, GA 30602; 5Institute of Bioinformatics, University of Georgia, Athens, GA 30602.
Abstract | Commonly found in commercial and backyard poultry productions, Coccidiosis, caused by Eimeria spp. presents a self-limiting intestinal infection based on the number of ingested oocysts. Intestinal replication of the parasite causes severe morphological alterations to the host`s gastrointestinal tract (GIT), marked, among others, by the disruption of the intestinal barrier. The objective of this study is to elucidate the dynamics of the intestinal barrier and parasite development, analyzing intestinal permeability elicited by Eimeria (E.) maxima infection and the ontogeny of lesion development throughout the first 10 days of infection. We herein demonstrate that infection with E. maxima leads to disruption of the intestinal barrier peaking at 6 days post infection (dpi). E. maxima down regulates mRNA expression of occludin and upregulates claudin-1 and JAM-2, indicating vasodilation, altered ion influx and intensive cell replication. This study also presents strong indicatives of a second peak of E. maxima replication following oocyst shedding, without reinfection.
Keywords | Intestinal barrier failure, Coccidiosis, Poultry, Eimeria, Tight junctions.
Editor | Muhammad Imran Rashid, Department of Parasitology, University of Veterinary and Animal Sciences, Lahore, Pakistan.
Received | June 26, 2019; Accepted | July 05, 2019; Published | September 20, 2019
*Correspondence | Samuel E Aggrey, Nutri Genomics Laboratory, Department of Poultry Science, University of Georgia, Athens, GA 30602, USA; Email: saggrey@uga.edu
Citation | Schneiders GH, Foutz JC, Milfort MC, Ghareeb AFA, Sorhue UG, Richter JN, Fuller AL, Williams SM, Rekaya R, Aggrey SE (2019). Ontogeny of intestinal permeability in chickens infected with eimeria maxima: implications for intestinal health. J. Adv. Parasitol. 6(3): 41-50.
DOI | http://dx.doi.org/10.17582/journal.jap/2019/6.3.41.50
Copyright © 2019 Aggrey et al. This is an open access article distributed under the Creative Commons Attribution License, which permits unrestricted use, distribution, and reproduction in any medium, provided the original work is properly cited.
Introduction
Coccidiosis is the most important infectious disease in the current poultry production systems, causing significant economic losses for the industry. The disease is caused by Apicomplexan parasites of the genus Eimeria spp. The coccidia`s life cycle is comprised of endogenous intracellular asexual replication followed by sexual development (McDougald and Jeffers, 1976). Eimeria (E.) maxima is one of the seven recognized species of coccidia that infect the chicken. The disease is marked by reduced growth, apathy, diarrhea and sometimes mortality. There is often emaciation, pallor, roughening of feathers and anorexia. Abundance of yellow-orange mucus and fluid in the distal portion of the jejunum and proximal portion of the ileum, edema, thickening and disruption of the mucosa and sometimes presence of blood in the intestinal lumen are observed at necropsy (McDougald and Fitz-Coy, 2013).
Due to the complex life cycle of Eimeria spp., it is not surprising that several components of the immune system play a role in mitigating the infection. While specific mechanisms such as cellular immunity, especially CD8+, are the most effective against coccidiosis (Trout and Lillehoj, 1996; Lillehoj, 1998; Zhang et al., 2016), non-specific barriers are of fundamental importance, especially in the early days in the course of infection and in the development of cellular immunity (Swaggerty et al., 2011; Lee et al., 2016). Non-specific factors include physical barriers, phagocytes, leukocytes and the complement system. Intestinal forms of the parasite such as meronts, gamonts and oocysts, cause severe morphological alterations to the host`s gastrointestinal tract (GIT), marked by the disruption of the intestinal barrier, distortion, rupture, segregation of adjacent cells, edema, cellular infiltration, sloughing of intestinal epithelia, resulting in the clinical signs observed in the disease (Cook, 1988; McDougald and Fitz-Coy, 2013; Zhang et al., 2016).
Tight junctions (TJ) are multi-protein complexes responsible for the regulation of permeability in the intestine via the modulation of its proteins (Sultana et al., 2013), by sealing the paracellular space between adjacent epithelial cells (Sander et al., 2005; Ulluwishewa et al., 2011). The main clinical sign of E. bovis infection in cattle is diarrhea, linked to the downregulation of TJ gene expression (Walker et al., 2015). Fluorescein isothiocyanate dextran (FITC-d) is 3000-5000 Da molecular weight and does not cross intact intestinal blood barrier in high quantities unless the intestinal barrier is compromised (Hamilton et al., 2015). Oral administration of FITC-d has been broadly used to assess the disruption of the intestinal barrier in chickens by measuring intestinal permeability (Zhang et al., 2016; Gilani et al., 2017). Similarly, the differential expression of TJ proteins such as zona occludens-1 protein (ZO-1), occludin, claudin 1 and junction adhesion molecule 2 (JAM-2) have been used to assess intestinal permeability in chickens (Chen et al., 2015; Li et al., 2015), however producing contrasting results.
The objective of this study is to elucidate the dynamics of the intestinal barrier and parasite development, analyzing intestinal permeability elicited by E. maxima infection and the ontogeny of lesion development throughout the first 10 days of infection, as well as investigating possible biomarkers for intestinal lesion in coccidiosis poultry models.
Materials and Methods
All experiments conducted in this study were performed under the Animal Use Proposal (AUP) A2015 04-005 approved by the Animal Care and Use Committee (IACUC) of the University of Georgia.
Single Oocyst Cloning
Freshly sporulated E. maxima oocysts from a North Carolina field strain were counted and suspended in PBS at a concentration of 500 oocysts/ml. Single oocysts were observed in 2 µl droplets under light microscopy and collected using a pipette. Twelve 14 days old-broilers kept in individual disinfected isolators were infected with one oocyst suspended in 200 µl of PBS via gavage. Fecal matter was collected from each isolator at 7 days post infection (dpi) and analyzed for the presence of oocysts by salt flotation. One volume of feces was solubilized in 9 volumes of saturated salt solution and the supernatant used to verify for the presence of oocysts in a McMaster chamber. Oocysts recovered from one of the chickens were sporulated as previously reported (You, 2014), and used to infect a second (p2) and third (p3) passages in chickens. The oocysts from the p3 were later sporulated and used for the experimental infections of chickens. The purity of the E. maxima clone was verified by PCR following published protocol (Jenkins et al., 2006).
Experimental Design
One hundred and ten 14-days old Ross708 broiler chickens were infected via gavage with 2x105 E. maxima sporulated oocysts suspended in water. Another 110 chickens were mock-infected with water (control). Five birds each from the infected and control groups were sampled daily for 10 days. On each day, the sampled chickens were gavaged with FITC-d for the assessment of intestinal permeability. Chickens were euthanized by cervical dislocation, followed by collection of ileum samples and assessment of lesion scores, microscopic lesion score and body weight. Throughout the course of the experiment, chickens were kept at 25˚C in batteries with wired floor, fed with broiler grower feed, with ad libitum access to water. Feces from underneath the cages were collected daily for the assessment of oocyst shedding by salt flotation.
Intestinal Permeability
Briefly, FITC-d was gavaged to the sampled chickens at 2.2 mg of FITC-d/bird. Two hours post administrations, blood was collected from the jugular vein and stored in tubes protected from the light and kept at room temperature for 3 hours to allow clotting, following with centrifugation (1000g for 15 min) to separate serum. Fluorescence levels of diluted serum (1:1 in PBS) were measured at an excitation wavelength of 485 nm and emission of 528 nm. FITC-d concentration per ml of serum was calculated based on a standard curve. Serum FITC-d levels were compared across infected and non-infected (control) chickens.
Intestinal Lesion Scores
Eimeria maxima specific lesions were scored daily according to Johnson and Reid (1970). Lesion scores ranged from 0 to 4, where 0 indicates no gross lesion scores, 1 indicates small amounts of orange mucus, 2 represents mid-intestine filled with orange mucus; 3, ballooned thickened intestines with caseous-looking content; and 4 indicates ballooned intestines with blood clots. For microscopic lesion scores, a 2.5 cm long portion of the jejunum, proximal to the Meckel’s diverticulum was opened and the mucosa scraped
Table 1: Sequences of real-time PCR primers
Target | Primer sequences | NCBI accession number | Source |
-Actin |
F: 5′ AGACATCAGGGTGTGATGGTTGGT 3′ R: 5′ TCCCAGTTGGTGACAATACCGTGT 3′ |
NM_205518.1 | (Habashy et al., 2017) |
Claudin-1 |
F: 5’ GGATGACCAGGTGAAGAAGATG 3’ R: 5’ GGGTGTGAAAGGGTCATAGAAG 3’ |
NM_001013611.2 | |
E. maxima |
F: 5’ TCGTTGCATTCGACAGATTC 3’ R: 5’ TAGCGACTGCTCAAGGGTTT 3’ |
M99058 | (Vrba et al., 2010) |
JAM-2 |
F: 5’ GCAACGCTGACTTCATTCTTAC 3’ R: 5’ CAATGCTTCGGCCTCAATTAC 3’ |
NM_001006257.1 | |
Occludin |
F: 5’ GAGCCCAGACTACCAAAGCAA 3’ R: 5’ GCTTGATGTGGAAGAGCTTGTTG 3’ |
NM 205,128 | (Wang et al., 2018) |
ZO-1 |
F: 5’ CACTAGAGGATGAGGAGGAAGA 3’ R: 5’ GGAATGGCTCCTTGTGGTATAA 3’ |
XM_015278981.2 |
JAM-2: Junction adhesion molecule 2; ZO-1: Zona occludens 1; F: forward primer; R: reverse primer.
with a dissecting knife. The scraping was placed on a slide and covered with a slide. 20 fields were examined at 1000x magnification and scored as follows: 0 (no oocyst present), 1 (1-10 oocysts per field), 2 (11-20 oocysts per field), 3 (21-30 oocysts per field), and 4 (more than 30 oocysts per field).
Oocyst Counts
Pooled fecal samples were homogenized and 5g of feces were dissolved in 50 ml of saturated salt solution, with 5 replicates per treatment. The solution was filtered in a coarse strainer to remove big particles. 1.5 ml of the filtered homogenized suspension was used to count E. maxima oocysts in a Macmaster chamber and expressed in oocysts per gram (opg).
Nucleic Acid Extraction
Tissue samples were stored in liquid nitrogen (-196˚C) immediately after collection for transportation purposes and at -86˚C in the laboratory. DNA was extracted using quick DNA Miniprep Kit (Zymo, Irvine, CA) following manufacturer`s guidelines, and stored at -86˚C. RNA was extracted using trizol. In brief, 100mg of frozen tissue were homogenized with 1ml of trizol, followed by phase separation with 0.2ml of chloroform, homogenization, incubation at room temperature (RT) for 3 minutes, centrifugation (12000 rpm, 15 min, 4˚C) and transferring 550µL of the aqueous phase into a tube for RNA precipitation with 0.5ml of isopropanol, homogenization and incubation (RT, 10 min), centrifugation (12000 rpm, 10 min, 4˚C), removal of the supernatant and wash of the pellet with 1ml of 75% ethanol, centrifugation (12,000 rpm, 5 min, 4˚C), removal of the supernatant, incubation (RT, 10 min), and dissolution of the RNA in 100µL of RNase free water followed by incubation (55-60˚C, 10 min) and storage of RNA at -80˚C for downstream applications. RNA was cleaned using RNeasy Mini Kit (Qiagen, Hilden, Germany), following manufacturer`s guidelines, and later reverse-transcribed high capacity cDNA reverse transcription Kit (Thermo Fischer Scientific, Waltham, MA).
qPCR
Real-time PCR reactions were performed on occludin, claudin-1, JAM2, ZO1 (Table 1) in a Step One Plus Real-time PCR machine (Applied Biosystems, Foster City, CA), in a 20µL reaction using 8.4µL of DNase-RNase free water, 0.3µL of each forward and reverse primer, 10µL of PowerUp SYBR Green Master Mix (Thermo Fischer Scientific, Waltham, MA) and 1µL of template DNA. True positive and negative controls for the reaction were included. The samples were tested in triplicates, and the relative amount of E. maxima DNA detected per sample was quantified using the 2-∆∆ct method (Livak and Schmittgen, 2001). The parasite load per sample was calculated from the amount of the E. maxima DNA (target) normalized to the amount of chicken β-actin (endogenous control). Cycling conditions were 50˚C for 2 min, 95˚C for 2 min, followed by 40 cycles at 95˚C for 15 seconds and 60˚C for 1 min and final melt curve of 95˚C for 15 seconds and 60˚C for 1 min.
Histological Analysis
Ileum samples were collected in a daily basis, fixed in 10% buffered formalin and embedded in paraffin. Tissue sections were cut and stained with hematoxylin and eosin (H&E) and analyzed microscopically.
Statistical Analysis
Statistical analysis and figures were generated with Prism 7.0 software (Graphpad Software Inc., San Diego, California). D’Agostino and Pearson test was applied to determine normality and determined whether data should be analyzed by parametric or non-parametric tests. Kruskal-Wallis test with multiple comparisons using Dunn’s method was used to compare non-parametric data. Parametric data were analyzed by one-way-ANOVA test with multiple comparison by Bonferroni’s method. All analyses were tested at the 5% level of significance.
Results
Body Weight Changes
The presentation disease was confirmed by the clinical signs shown by the chickens, consisting of apathy, aqueous diarrhea, reduced feed intake and dehydration. Daily body weight is shown in Figure 1a. While there are no significant changes in body weight between infected and control birds from 1 to 6 dpi (p ≥ 0.07), infection with E. maxima significantly reduced body weight at 7 (522.1 ± 119.8g vs 663.7 ± 69.5g; p = 0.0010) and 8 dpi (509.8 ± 144.2 vs 800.8 ± 135.7g; p < 0.0001). Although infected chickens presented lower body weight at 9 dpi (696.4g ± 79.7g) as compared to the control counterparts (783.5 ± 157.2g), this difference was not statistically significant (p = 0.1583). At 10 dpi, infected chickens presented significant lower weight gain (730.3 ± 129.8g) as compared to uninfected controls (840.4 ± 167.5g; p = 0.0022).
The Peak of Intestinal Lesions Precedes Oocyst Shedding
Uninfected chickens did not shed oocysts throughout the experiment. While there was no shedding of oocysts from infected chickens from 1 to 5 dpi. Shedding was significantly higher at 6 dpi (15753.4 ± 9083.0 opg; p = 0.0006) and 7 dpi (p < 0.0001), with an average of 64,068.46 ± 38,635.06 opg at the peak of shedding (7 dpi). Although infected chickens shed oocysts 8 dpi (7,434.09 ± 5,529.37; p = 0.5435), 9 dpi (424.46 ± 1342.10; p > 0.9999) and 10 dpi (967.20 ± 2805.91; p > 0.9999), this was non-statistically significant as compared to zero (p = 0.5435; Figure 1b). E. maxima-specific intestinal lesions were scored and results are depicted in Figure 1c. While uninfected control chickens did not present lesions characteristic of infection (score 0) throughout the experiment, infected chickens presented significant higher lesion scores from 4 to 7 dpi (p ≤ 0.0116), with peak at 6 dpi (p < 0.0001) and respective average score of 3.6 ± 0.55. Following the same trend, micro-scores, marked by the quantification of E. maxima developmental stages in intestinal scrapings (Figure 1d), where significantly higher in infected chickens from 2 to 7 dpi (p ≤ 0.0161), with peak at 6 dpi and respective average lesion score of 4 ± 0 (p < 0.0001). Micro-scores where not statistically different at 8 and 9 dpi (p > 0.9999), however, infected chickens presented a significantly higher micro-score at 10 dpi (0.8 ± 0.84; p= 0.0161). Contrarily, no stages of E. maxima were found in intestinal scrapings from uninfected control chickens (micro-score of 0) throughout the experiment.
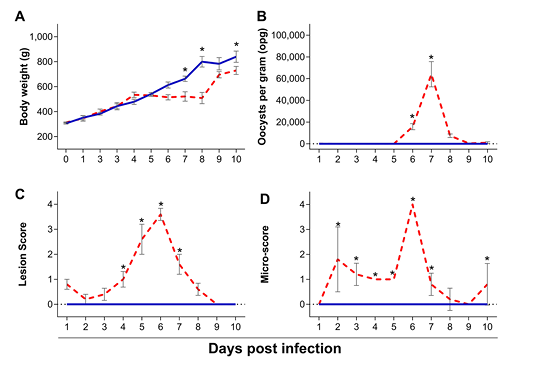
Figure 1: Body weight (A), oocyst shedding (B), lesion scores (C) and microscopic scores (D) of chickens infected with Eimeria maxima (red dashed line) as compared to uninfected chickens (blue solid line), assessed from 1 to 10 days post infection (dpi). Standard error means (SEM) are depicted by vertical lines. Two-way-ANOVA test was performed at 5% level of significance (p < 0.05), with multiple comparisons corrected by Bonferroni’s method. Significant differences between the groups are indicated by the superscript asterisk.
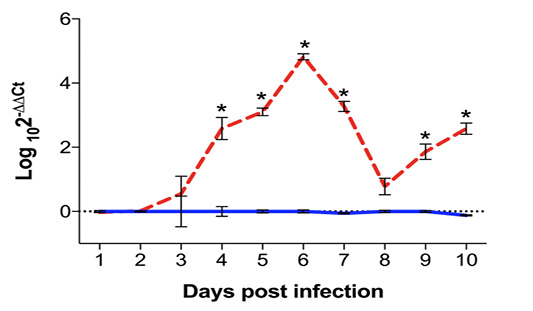
Figure 2: Quantification of Eimeria maxima DNA in ileum samples of infected (red dashed line) and uninfected (blue solid line) chickens, assessed by qPCR from 1 to 10 days post infection (dpi) and expressed as Log102-∆∆Ct. Standard error means (SEM) are depicted by vertical lines. Two-way-ANOVA test was performed at 5% level of significance (p < 0.05), with multiple comparisons corrected by Bonferroni’s method. Significant differences between the groups are indicated by the superscript asterisk.
Eimeria Maxima Presents Two Peaks of Intestinal Replication
The quantification of E. maxima DNA in ileum sections was performed through qPCR, and normalized by the presence of chicken β-actin DNA in the sample. Results, expressed as log102-∆∆Ct are expressed in Figure 2. While there was no detection of E. maxima DNA in samples from uninfected control chickens, parasite load in the samples started to increase at 3 dpi, and was significantly higher from 4 to 7 dpi (p < 0.0001) with peak at 7 dpi (p < 0.0001) and respective average load of Log103.27 ± 0.62. The presence of E. maxima DNA was not statistically significant at 8 dpi in infected chickens as compared to uninfected controls (Log100.775 ± 1.00 vs Log106.67x10-11 ± 0.13; p = 0.0972). However, there was another significant increase in Eimeria load starting at 9 dpi (Log101.83 ± 0.94 vs Log106.67x10-11 ± 0.11; p < 0.0001) and continuing at 10 dpi (Log102.58 ± 0.68 vs Log10-0.123 ± 0.06; p < 0.0001).
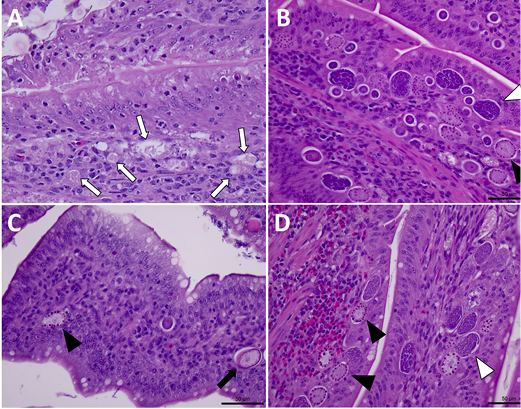
Figure 3: Detection of E. maxima in histological samples of intestinal sections. (A) visualization of schizonts (white arrows) at 4 dpi, 200x. (B) Visualization of macrogametocytes (black arrowheads) and microgametocytes (white arrowheads) at 5 dpi, 400x. (C) Visualization of macrogametocyte (black arrowhead) and immature oocyst (black arrow) at 6 dpi, 400x. (D) Visualization of macrogametocytes (black arrowheads) and microgametocytes (white arrowheads) at 7 dpi, 400x). Tissues were stained with hematoxylin and eosin (H&E), slides analyzed by optical microscopy.
Histological Sections Reveal the Continued Asexual and Sexual Replication in the Small Intestine Following Oocyst Shedding
Sections of the small intestine where fixed, stained by H&E and analyzed microscopically (Figures 3 and 4). There was no histological evidence of coccidia infection in sections from uninfected control chickens throughout the experiment. The first developmental stages of E. maxima were detected in intestinal tissue samples from infected chickens at 4 dpi. These were accompanied by the histological signs of coccidial enteritis, characterized by GALT hyperplasia in the lamina propria (Figure 3a). At 5 dpi, sexual stages of E. maxima were first observed in moderate numbers (Figure 3b). At 6 dpi moderate to severe numbers of E. maxima developmental stages were observed, including oocysts and macrogametocytes (Figure 3c). At 7 dpi, small intestines presented marked multifocal corrosive enteritis with hemorrhage, and the presence of oocysts and sexual stages of E. maxima were observed in rare to moderate numbers (Figure 3d). At 8 (Figure 4a), 9 (Figure 4b) and 10 (Figure 4c) dpi, sections evidenced the presence of sexual stages of development of E. maxima in mild to moderate numbers.
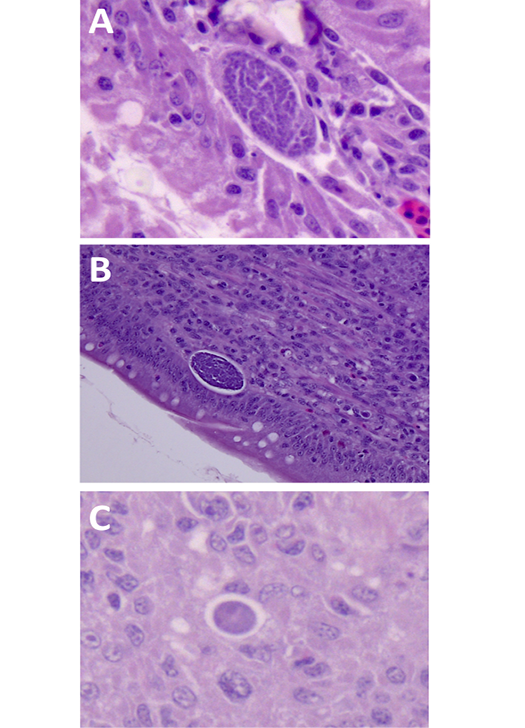
Figure 4: Detection of E. maxima in histological samples of intestinal sections. (A) visualization of microgametocyte at 8 dpi, 630x. (B) Visualization of microgametocyte at 9 dpi, 400x. (C) Visualization of macrogametocyte immature macrogametocyte at 10 dpi, 630x. (D) Tissues were stained with hematoxylin and eosin (H&E), slides analyzed by optical microscopy.
Disruption of the Intestinal Barrier
The assessment of intestinal barrier disruption was done through presence of FITC-d in the serum, and results are shown in Figure 5. Intestinal permeability was significantly higher at 5 and 6 dpi (p < 0.0001). At 6 dpi, the peak of intestinal barrier disruption, the presence of FITC-d in the serum of infected chickens averaged 433.47± 147.43 ng/ml, significantly higher as compared to the average of FITC-d in the serum of uninfected controls (339.38 ± 34.15 ng/ml; p < 0.0001). The concentration of FITC-d in the serum of infected chickens was not significantly different as compared to the control group at 7 to 10 dpi (p ≥ 0.7391).
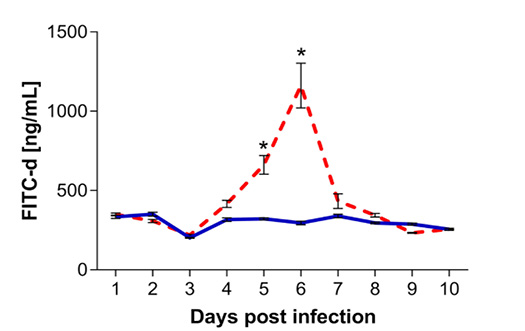
Figure 5: Quantification of fluorescein isothiocyanate dextran (FITC-d) in serum samples collected from chickens infected with Eimeria maxima (red dashed line) as compared to uninfected chickens (blue solid line). Presence of FITC-d was assessed by ELISA from 1 to 10 days post infection (dpi). Standard error means (SEM) are depicted by vertical lines. Two-way-ANOVA test was performed at 5% level of significance (p < 0.05), with multiple comparisons corrected by Bonferroni’s method. Significant differences between the groups are indicated by the superscript asterisk.
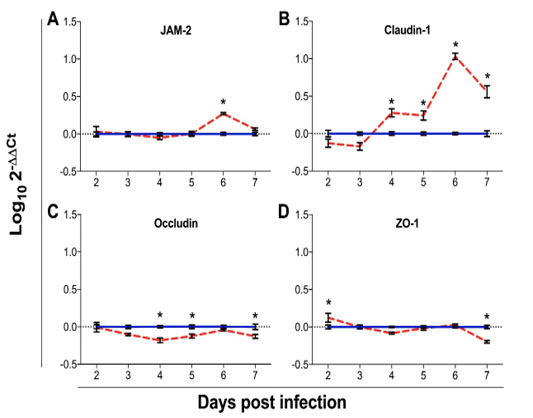
Figure 6: Gene expression analysis of the tight junction genes: junction adhesion molecule 2 (JAM-2; A), claudin 1 (B), occludin (C) and Zona occludens 1 (ZO-1; D) in chickens infected with Eimeria maxima (red dashed line) as compared to uninfected chickens (blue solid line). Data were assessed from 2 to 7 days post infection (dpi). Expression of mRNA was done by qPCR, with results expressed as Log102-∆∆Ct. Standard error means (SEM) are depicted by vertical lines. Two-way-ANOVA test was performed at 5% level of significance (p < 0.05), with multiple comparisons corrected by Bonferroni’s method. Significant differences between the groups are indicated by the superscript asterisk.
The expression of TJ genes was assessed from 2 to 7 dpi (Figure 6). The expression of JAM-2 (Figure 4a) was not statistically significant from 2 to 5 dpi (p > 0.9999) and at 7 dpi (p = 0.8369), however, at 6 dpi, infected chickens presented significantly higher mean mRNA expression of JAM-2 (Log100.27 ± 0.06; p < 0.0001) as compared to the mean expression of uninfected chickens (Log101.33x10-10 ± 0.08). While the expression of claudin-1 was non-statistically significant altered from 2 to 3 dpi (p ≥ 0.0598), it was significantly higher from 4 to 7 dpi (p ≤ 0.0018), with peak at 6 dpi (Log101.03 ± 0.17; p < 0.0001), as compared to control chickens (zero). Changes in the mRNA expression of occludin were not significant at 2 to 3 (p ≥ 0.1151) and at 6 dpi (p > 0.9999), however, the expression of occludin was significantly reduced in infected chickens at 4 (Log10-0.18 ± 0.13 vs Log106.67x10-11 ± 0.07; p = 0.0003), 5 (Log10-0.13 ± 0.11 vs Log106.67x10-11 ± 0.09; p = 0.0261) and 7 dpi (Log10-0.13 ± 0.11; p = 0.0229), as compared to the control counterparts. While the expression of ZO-1 gene was slightly elevated at 2 dpi in infected chickens (Log100.13 ± 0.23 vs. Log10-1.89x10-18 ± 0.10; p = 0.0031), there were no statistically significant differences between the groups from 3 to 6 dpi (p ≥ 0.1301). However, there was a significantly lower expression of ZO-1 mRNA in infected chickens at 7 dpi (Log10-0.197 ± 0.07; p < 0.0001) as compared to the control group (Log106.67x10-11 ± 0.09).
Discussion
The first line of defense against Eimeria spp. is provided by the infected epithelial cells and the cells in close contact with them, such as intra-epithelial lymphocytes and fibroblasts (Laurent et al., 2001). The maintenance of intestinal integrity is a fundamental aspect for animal health and productivity, since it functions as a nutrient absorption system and as a barrier, preventing systemic infections (Neish, 2002), therefore preventing the incidence of enteric diseases, improving performance and reducing mortality (Droleskey et al., 1994). In poultry, Eimeria spp. replicates in the intestine causing extensive tissue damage. E. maxima causes congestion and edema, cellular infiltration, thickening of the mucosa, with the later generations of schizonts and sexual stages developing deeper in the tissues, causing considerable disruption of the mucosa (McDougald and Fitz-Coy, 2013). The current study was undertaken to elucidate the dynamics of intestinal barrier disruption as well as parasite development during the course of infection of E. maxima in broiler chickens.
The minimum prepatent period for E. maxima reported is between 5 (Long and Reid, 1982) and 6 dpi (Long, 1959), with typical peak of oocyst shedding at 7 dpi (Cha et al., 2018). The strain of E. maxima used on this study has a prepatent period of 6 dpi and peak of oocyst shedding in the feces at 7 dpi, corroborating with previous reports in the literature. The development of E. maxima within the host was assessed at the histological level in sections of the small intestine. The presence of oocysts and sexual stages of the parasite peaked at 6 dpi in the intestinal wall, and at 7 dpi in the feces. Only mild to moderate numbers of sexual stages of the parasite were observed at 7 to 10 dpi.
Previous studies have reported the sequential decay in oocyst shedding following 7 dpi with E. maxima (Idris et al., 1997; Al-Badri and Barta, 2012; Cha et al., 2018). Although non-statistically significant, we observed that oocyst shedding showed a small trend to resume sequential increase at 9 and 10 dpi, indicating a possible restart in oocyst production. This phenomena has not been reported in previous studies, but the continuous presence of oocysts in the intestinal wall following the patent period has been reported and suggested to play some part in the crowding effect of E. maxima (Williams, 2001). In the current study, there was a significant increase of developmental stages in intestinal scrapings at 10 dpi and in the detection of E. maxima DNA concentration in ileum sections at 9 and 10 dpi, corroborating the oocyst shedding. Altogether, these data indicate the possible recommence of the infection cycle of E. maxima following the first round of oocyst shedding in chickens without reinfection. A study conducted by Dubey and Jenkins (2018) describes the formation of fifth generation schizonts at 5 dpi, after the formation of gamonts, supporting the hypothesis that E. maxima can continue its replication past the first round of oocyst shedding without reinfection. The possibility of reinfection in this study was minimized be the housing of chickens in wired floor cages and daily removal of waste.
Eimeria spp. is entirely dependent on the survival and continued existence of the parasitized epithelial cell for a definite length of time, therefore, changes in the cell population kinetics of the intestinal epithelium, associated with other morphological changes, are important factors in the outcome of infection (Fernando and McCraw, 1973). Coccidia infection causes villous atrophy, which leads to reduction of functional epithelial cells available for parasite development (Pout, 1967). Similar changes are believed to occur during E. maxima infection, raising the possibility that developmental stages reminiscent in the intestine could take advantage of the compensatory growth of new epithelial cells to resume their development, giving rise to the second peak of replication. The reduction in cell availability has been considered as a factor equally important, if not more, than the crowding effect in the self-limiting nature of coccidiosis in poultry (Williams, 2001). Further studies should address the importance of the infectious dose in the continued replication of E. maxima.
Integrity of the intestinal barrier has been traditionally assessed in vivo by the presence of FITC-d in the serum of chickens following gavage administration of the substance suspended in water. Studies utilizing gene expression as biomarkers to determine intestinal integrity demonstrate a high level of variability in which genes are reliable indicators of intestinal barrier dysfunction, therefore FITC-d administration continues to be the primary marker for intestinal barrier integrity in chickens (Chen et al., 2015; Gilani et al., 2018). The peak of damage to the intestinal epithelia reported here was at 6 dpi, as demonstrated by the circulatory levels of FITC-d. This correlates with the highest presence of E. maxima sexual stages and oocyst maturation in the epithelia demonstrated by histology and qPCR. Sexual stages of E. maxima develop in deeper tissues between 5-8 dpi, an in severe infections cause considerable disruption of the mucosa (McDougald and Fitz-Coy, 2013). Based on the quantification of FITC-d in the serum, the intestinal wall shows the first signs of repair at 7 dpi, prior to the possible relapse of infection.
Reduced intestinal barrier function can result in increased bacterial translocation and inflammatory responses, bacterial chondonecrosis with osteomyelitis, and necrotic enteritis (Teirlynck et al., 2011; Wideman and Prisby, 2012; Latorre et al., 2014; Tellez et al., 2014; Rodgers et al., 2015). Tight junctions (TJ) are multi-protein complexes which are crucial for the integrity and function of the epithelial barrier, as they exert a dual function linking cells and forming channels that allow permeation between cells, resulting in epithelial surfaces with different tightness (Awad et al., 2017).
JAM-2 is primarily expressed in endothelial cells, where it plays a dual role in the adhesion and transmigration of lymphocytes and regulation of vascular permeability (Johnson-Leger et al., 2002). It has also been reported that permeability of Mardin-Derby Canine Kidney (MDCK) cells increases upon JAM-2 expression (Aurrand-Lions et al., 2001), evidencing its role in favoring cellular permeability. Our results show the significant upregulation of JAM-2 mRNA in infected chickens at 6 dpi, demonstrating the augmented vascular permeability caused by E. maxima infection. These disagree with previous report in which there was no differential expression of JAM-2 in chickens with intestinal barrier failure as compared to chickens with intact intestinal barrier (Chen et al., 2015). However, the concomitant infection by more than one Eimeria spp. and utilization of non-starch polysaccharides in the aforementioned study should be considered as possible factors involved on this difference.
Claudin-1 is a member of multiple-span transmembrane proteins called claudins and classified as a “tight” or “sealing claudin”, as opposed to the pore forming “leaky” claudins (Garcia-Hernandez et al., 2017). Upregulation of claudin-1 expression has been observed as a response to inflammation associated with a leaky epithelial barrier (Weber et al., 2008), where claudin proteins have been proposed to actively contribute to disease pathogenesis. Increase in claudin-1 expression has also been associated with enhanced cellular proliferation (Huang et al., 2015). In response to inflammation, altered claudin protein profiles in the TJ are associated with perturbed paracellular movement of fluid and solutes, which is in turn reflected in overall change in epithelial barrier function (Garcia-Hernandez et al., 2017). This study demonstrates the upregulation of claudin-1 at the peak of intestinal lesions caused by E. maxima, indicating a compensatory gene expression in response to the accentuated intestinal permeability.
Occludin is involved in the regulation of inter-membrane diffusion and paracellular diffusion of small molecules (Saleh and Elson, 2011), and is down-regulated in humans with inflammatory bowel diseases (Assimakopoulos et al., 2011; Saleh and Elson, 2011). The downregulation of occludin has been reported in the intestinal barrier function model developed by Chen et al. (2015), by combining infection with E. acervulina, E. maxima and E. tenella with non-starch polysaccharides in broiler chickens. Our results demonstrate no significant variation in occludin mRNA expression at 6 dpi. On the other hand, there is significant downregulation of this gene at 4 and 5 dpi, prior to the peak of E. maxima intestinal replication, and again at 7 dpi. This possibly indicates the downregulation of this gene at the lowest levels of parasite replication only. The significant downregulation of occludin at 7 dpi also supports our data demonstrating the recommence of a second cycle of infection. The expression levels of occludin have been shown to be inversely correlated with the translocation of FITC-d from the GIT to the blood (Cani et al., 2009), and movement occludin from the TJ into the cytoplasmic vesicles occurs frequently during barrier function loss (Shen et al., 2008).
ZO-1 is a plaque protein that acts as adaptor to connect transmembrane proteins to the perijunctional actomyosin ring (Ulluwishewa et al., 2011), and plays a major role in the formation of TJ in epithelial cells (Tsukita et al., 2001). Our results demonstrate minimal differences in mRNA expression of ZO-1 between infected and non-infected chickens, indicating that E. maxima infection does not severely affect cytoskeleton rearrangement.
Intestinal health is a crucial factor in animal production, and is closely related to well-being, feed intake and the efficient absorption of nutrients. In poultry, intensive selection for higher weight gain and lower feed conversion ratio has produced breeds that have extremely high feed intake as one of their main characteristics. Among other factors, intestinal infection caused by Eimeria spp. severely damages the intestinal integrity, leading to intestinal lesions, malabsorption, secondary infections, and dysbiosis. We herein show that infection of chickens with E. maxima results in the differential expression of TJ genes resulting in increased vascular permeability and cellular influx. Changes in the molecular structure of the junctional complexes results in decreased absorption of nutrients, increased secretory passage of ions and water, causing leak flux diarrhea and augmented passage of macromolecules from the lumen, inducing intestinal inflammation (Barmeyer et al., 2015). The differential expression of TJ genes is a valuable tool that can be further developed with the objective of monitoring intestinal health in poultry. It is, however, unlikely that a single biomarker will be sufficient to track all the aspects of intestinal health and deficiencies thereof, hence, combination of multiple biomarkers is the most promising way of tracking intestinal health in a holistic way in the future.
In summary our results indicate that infection with E. maxima leads to disruption of the intestinal barrier by downregulation of the expression of occludin and upregulation of claudin-1 and JAM-2. Further studies should address other TJ genes in order to provide a more holistic view of the intestinal barrier dysfunction during coccidiosis. Additional data in the expression of TJ genes should provide deeper understanding of the assessment of efficacy of new methods of therapy and control of coccidiosis in poultry. It is important to consider that other Eimeria spp. will likely provide somehow different patterns of dysfunction, based on the cycle and possibly on the infectious dose. We also demonstrate herein a strong indication of a second peak of E. maxima replication in the intestines. To our knowledge, this fact has not been previously reported, although previous literature provides strong indicatives of this phenomena. Further research is necessary to prove if intestinal cell availability could be the cause of such observation.
Funding
This work was partially supported by a cooperative grant 58-6040-8-034 from the United State Department of Agriculture-Agricultural Research Service. The funder had no role in the study design and implementation, data collection and analysis, decision to publish or preparation of this manuscript.
Acknowledgements
The Ross708 chicks were donated by Danny Goyne-Aviagen, Inc., Albertville, AL, USA.
Conflict of interest
The authors declare that there is no conflict of interest.
Authors Contribution
The experiment was conceived and planned by GHS, ALF, RR and SEA. It was executed by GHS, JCF, AFAG, JNR, UGS, SMW, MCM. Draft manuscript was prepared by GHS and edited by ALF, SMW, RR and SEA.
References